Microscopes – observing living organisms in order to fight disease
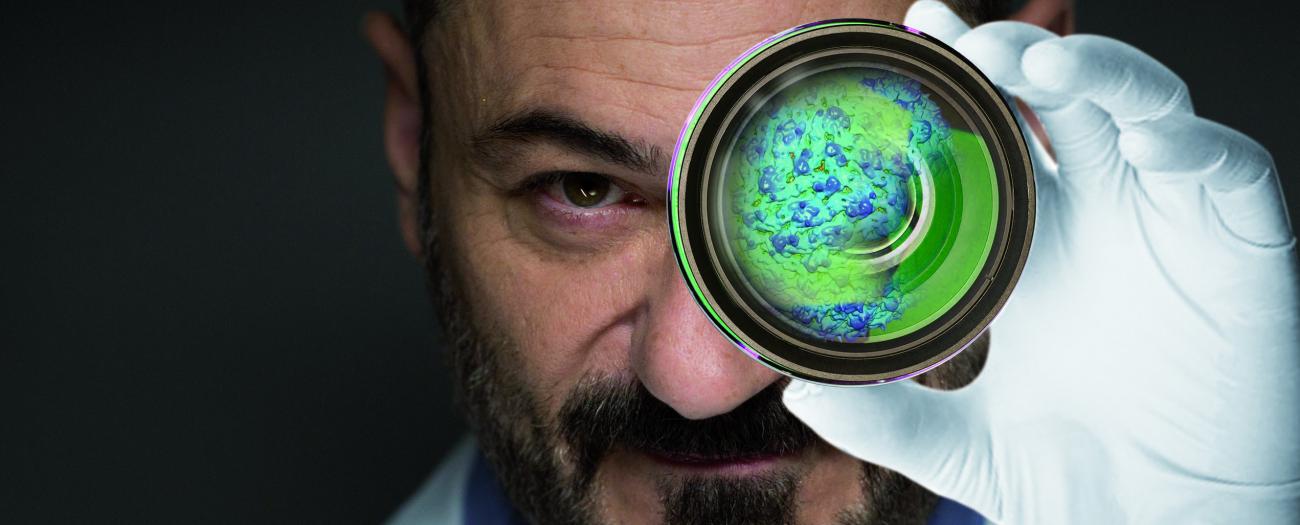
With the naked eye, humans can make out a detail measuring roughly 1 mm from a distance of 3 meters. Titan Krios™, the world's most powerful microscope, which will be officially inaugurated in July 2018 at the Institut Pasteur, magnifies objects millions of times (compared with just 10x for the earliest microscopes) and reveals what is happening at the atomic level. Scientists investigating diseases now have access to an entire range of highly sophisticated microscopes, which are often an essential prerequisite for their research. But before we look at those, we need to go back to when the infinitely small was first conquered to understand the extraordinary development of microscopes.
A new world is revealed
Dutch opticians Janssen and son are said to have developed the first microscope in 1595 by combining two glass lenses (one an objective and one an eyepiece). This was before the first mention of the term, which denotes any instrument used to see elements that are invisible to the naked eye. It comes from the Greek mikros (small) and skopein (to look). But the true fathers of microscopy in biology are unquestionably the Englishman Robert Hooke and Dutchman Antoni Van Leeuwenhoek. Thanks to his microscope with three objective lenses, Hooke made many observations (insect wings, fleas, lice, etc.) that he drew, annotated and compiled in his book Micrographia, published in 1667. It was the first documented work of the microscopic world. We have him to thank for the term "cell" – the basic unit of life (our body contains 10,000 billion of them!) – given to the small sac-like structures that he made out on cork slides.
"Animalcules" under the microscope
Shortly after, Van Leeuwenhoek made much more powerful microscopes with magnification up to 200x (compared with 20x for Hooke's) and he observed a number of elements, including rain drops, dental plaque, sections of intestine and mussels. He is famous for having been the first to observe bacteria, which he named "animalcules", and reported his discovery to the Royal Society of London in 1673. Two centuries later, Louis Pasteur proved that "animalcules" caused disease and the first pathogenic bacteria were identified under the microscope, i.e. the tuberculosis bacillus by the German Robert Koch in 1882, and the plague bacillus by Pasteurian Alexandre Yersin in 1894. But infectious agents such as viruses – a thousand times smaller than bacteria – remained invisible. So Louis Pasteur developed the rabies vaccine knowing that a transmissible agent was responsible but without ever seeing it (the virus was only observed in 1962).
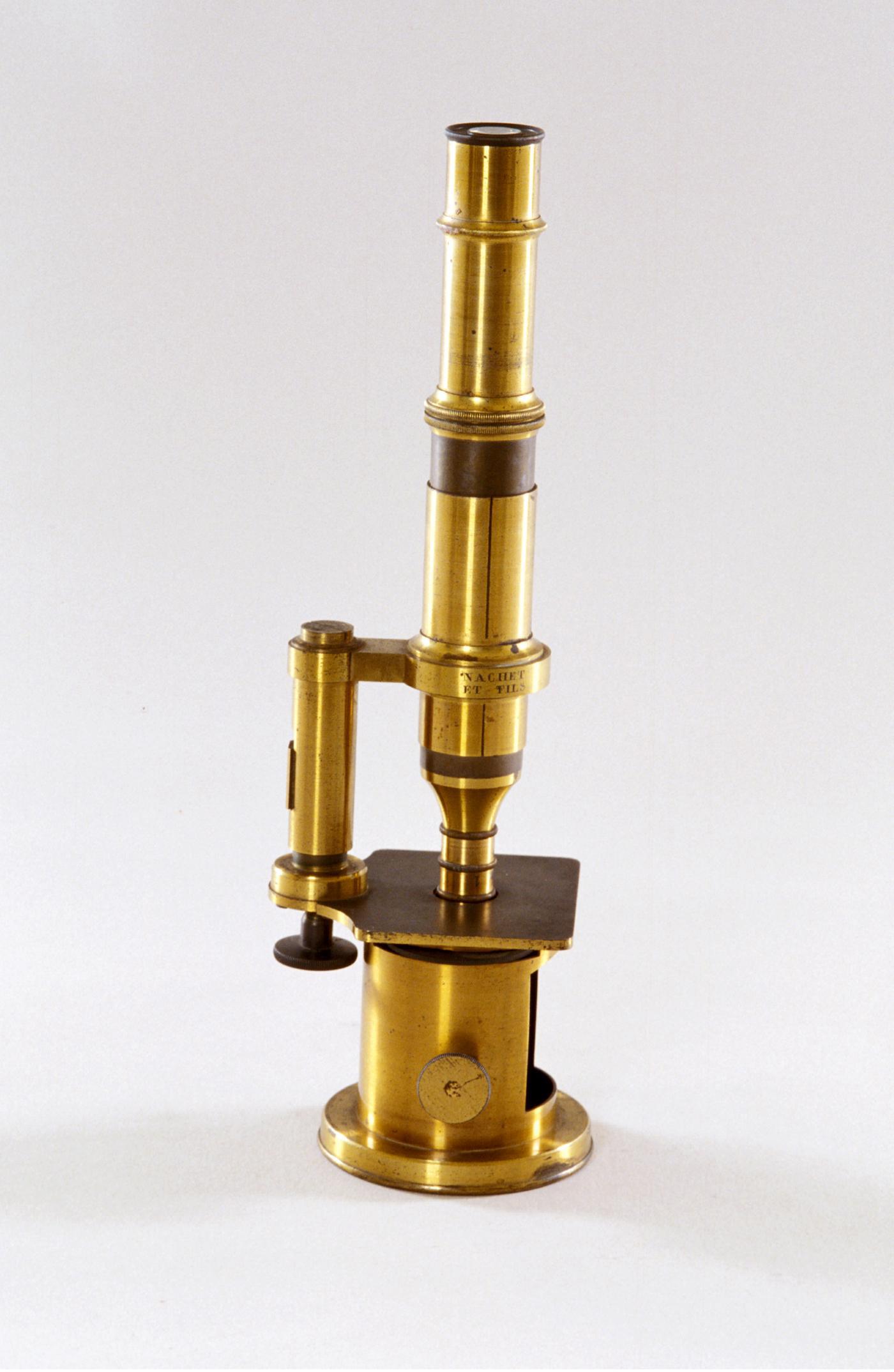
Optical microscopy and electron microscopy
To observe viruses, scientists had to go right down to nanometer level (i.e. a billionth of a meter or 50,000 times smaller than the diameter of a human hair) – the influenza virus, for example, measures roughly 100 nanometers –, and wait for the second half of the 20th century and the arrival of electron microscopes, the first prototypes of which were built by German engineers in the early 1930s. Instead of using light (made up of photons) and glass lenses, they came up with the idea of using much smaller electrons, accelerated in vacuum through cathode ray tubes, and electromagnetic lenses. This meant that they could observe elements even smaller than those visible with optical microscopes.
AIDS and Zika – hunting viruses using images
"The microscopic world is amazing!" exclaims Olivier Schwartz, Head of the Virus and Immunity Unit at the Institut Pasteur. "Its images help to illustrate or even fuel our discoveries. To study interactions between viruses and cells in the body, I have always used different microscopy techniques and I've witnessed the incredible progress made over the last few years, whether in optical microscopy, which is perfect for observing cells, or electron microscopy, which is mainly used for seeing viruses. Today, we combine both, like Google Maps does. We can get an overall view – and observe infected and non-infected cells for example – then look at the finer details, right down to viral particles on a cell membrane." Olivier Schwartz's team was behind major discoveries concerning the "relationships" between the AIDS virus and its target cells (CD4 T lymphocytes, see image) and is also looking closely at emerging viruses, such as chikungunya or Zika. "Time-lapse microscopy enables us to film the action of fluorescent-tagged viruses in cells in real time, and we have therefore recently been able to observe and describe the implosion of cells infected with the Zika* virus, which may be a defense mechanism used by the body to eliminate the virus. We are looking into this." On the researcher's desk, close to a small collection of vintage microscopes, there is a strange white structure – three mandarin-sized spheres joined by "arms". Thanks to a confocal microscopy image and a 3D printer, he can today hold these initially-real "communicating" cells in his hands, turn them around and see them with his own eyes. There is no end to the progress made!
See the Lettre de l’Institut Pasteur no.98. (page in French)
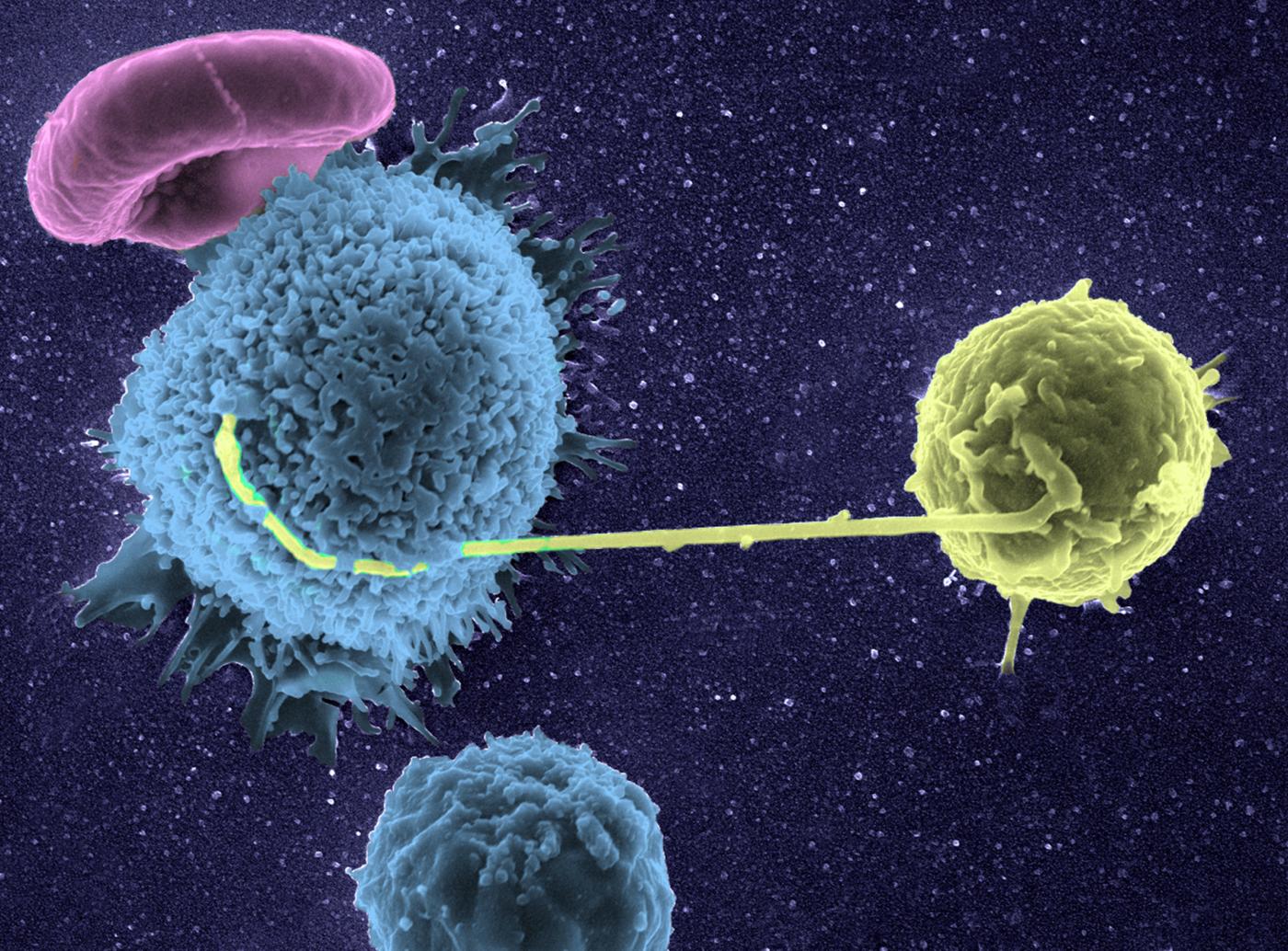
Every experiment has its microscope
But electron microscopes can only be used to observe elements set in ultra-thin sections (roughly fifty nanometers) and "fixed" with chemical elements or the cold (cryogenics). Only optical microscopes can be used to examine living elements (in cells in culture or experimental models), and therefore observe pathological phenomena in real-time – bacterial invasion of tissue, movement of metastatic cells, etc. – and even film them (using time-lapse microscopy). Instruments have become specialized with transmission electron microscopes, scanning electron microscopes (to see in relief – watch the video below), confocal microscopes (to see in 3D) and two-photon microscopes (to see in depth). Every experiment has its microscope, and biologists often use several types of microscope to obtain overall and more detailed views (see below), as correlative microscopy approaches link the different levels of observation. But advances in microscopy do not only depend on improvements in the instruments themselves.
What is a scanning electron microscope?
A video from the French youtuber "e-penser" (Bruce Benamran).
Meningitis – track the bacteria in our vessels
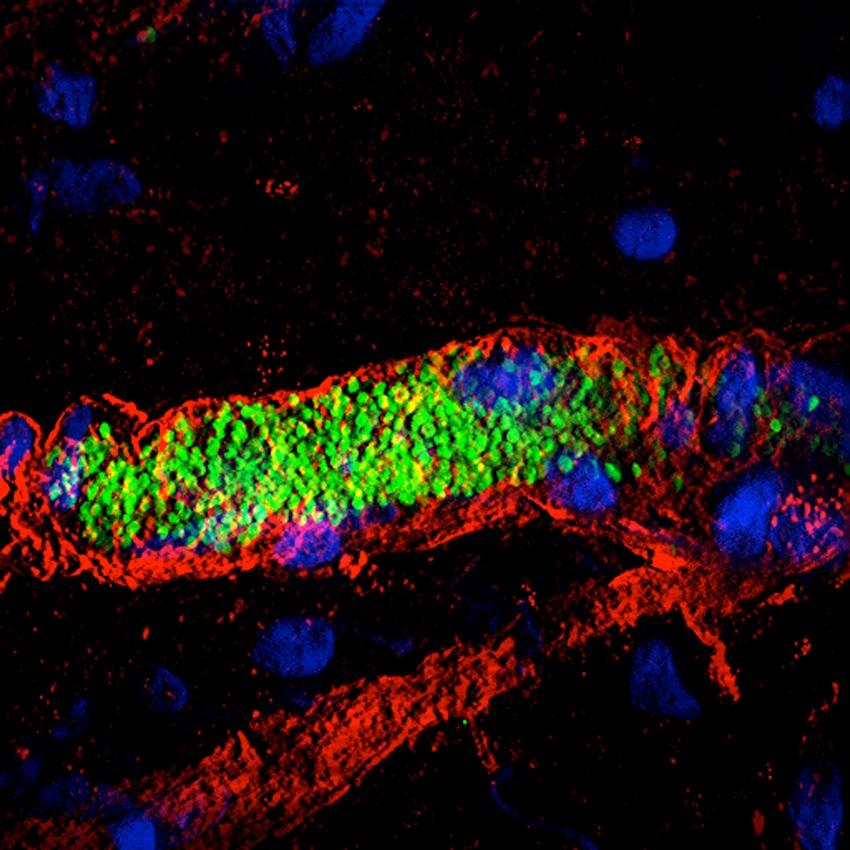
"We are studying a key step in infection by meningococci (responsible for serious meningitis and septicemia), i.e. the ability of these bacteria to interact with blood vessels and adhere to them", explains Guillaume Dumenil, Head of the Pathogenesis of Vascular Infections Unit at the Institut Pasteur. "These bacteria cluster together and multiply in small vessels until they block them. The vessels then end up bursting, compromising blood flow during septicemia and releasing bacteria in the cerebrospinal fluid during meningitis. We are observing this "vascular colonization" in real time on experimental models, thanks to microscopy apparatus set up on a shock-proof table and under a hood to prevent contamination*. In addition, we are studying sections of tissue in incredible detail using an electron microscope from the Ultrastructural BioImaging Unit (see p…). A fluorescence microscope has also enabled us to see how bacteria wrap themselves in the membrane of vessel wall cells to latch on. By combining all these techniques, we get a clear idea of what is happening in the body." The aim? To identify the vessel adhesion factors used by meningococci, as they are potential therapeutic targets.
*Cost of the apparatus: €500,000.
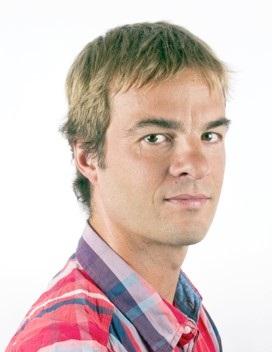
Guillaume Dumenil, Head of the Pathogenesis of Vascular Infections Unit at the Institut Pasteur.
We are studying a key step in infection by meningococci (responsible for serious meningitis and septicemia), i.e. the ability of these bacteria to interact with blood vessels and adhere to them.
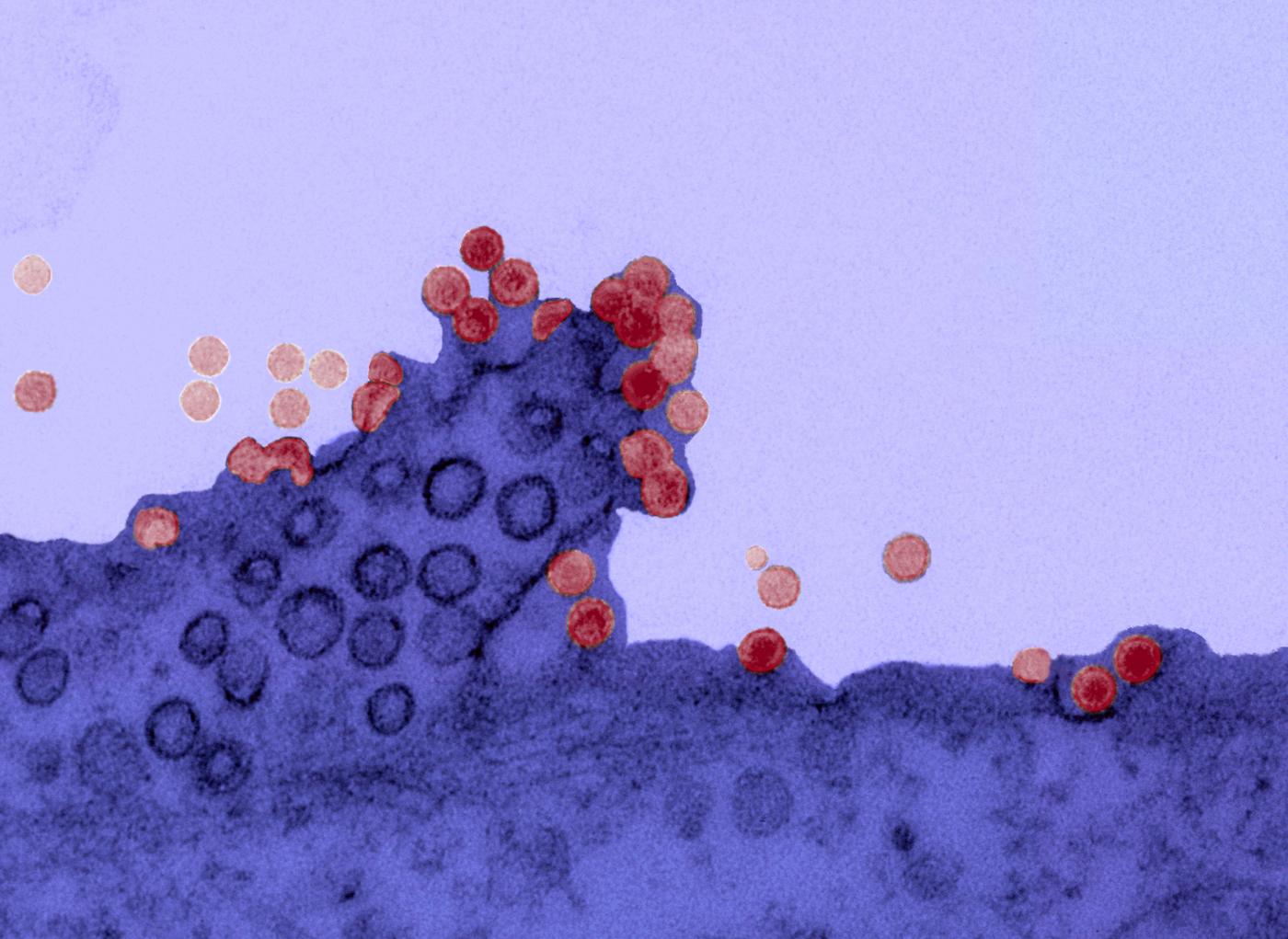
Chikungunya virus on the surface of a cell. Copyright: Institut Pasteur.
The "green revolution" in microscopy
A major breakthrough came with the discovery of a green fluorescent protein, or GFP, in jelly fish in 1962. When genetically coupled to a protein that scientists wish to study, the GFP "marks" it like a fluorescent label. Thanks to this molecular "telltale" and a fluorescence microscope, the activity of a biomolecule can be "highlighted" in green in its natural environment, without its function being impaired (the "fluorochromes" used to mark molecules in the past were toxic for the cell). It is possible to "tag" cells, bacteria or viruses, follow their journey in the body, and see when and where a given protein is produced and in what quantity (according to fluorescence intensity). GFP launched a real "green" revolution in microscopy in the 1990s and the three scientists that initiated the technique were awarded the 2008 Nobel Prize in Chemistry. One of them managed to modify GFP to obtain fluorescent proteins in other colors (red, blue, etc.), and therefore mark different elements at the same time. The revolution turned rainbow color… and GFP is now routinely used in a great many laboratories throughout the world.
Brain tumors – the zebrafish under the microscope
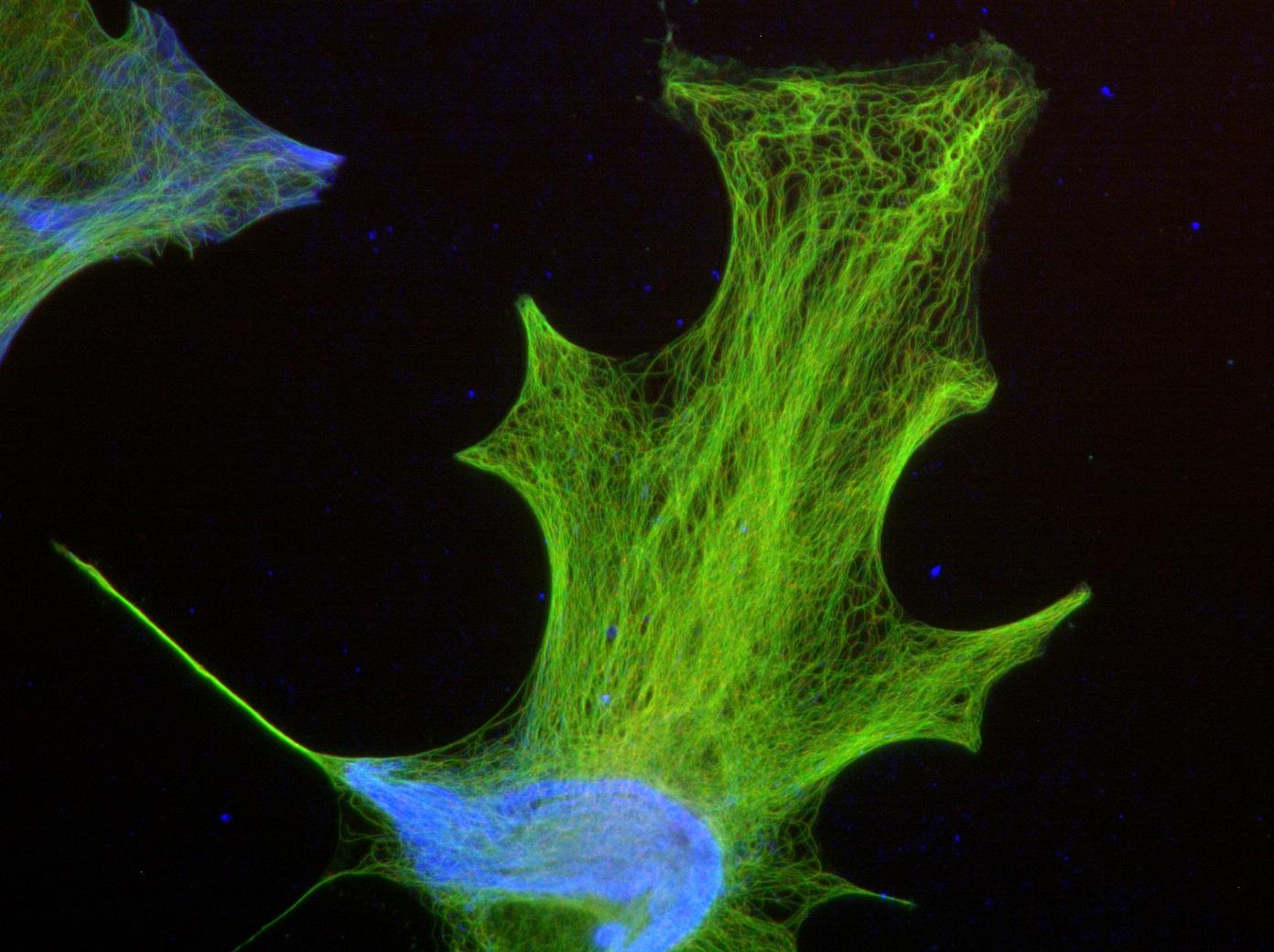
The zebrafish, a small tropical fish (which when fully grown measures no more than 5 cm), has become a research model for many biologists. Many choose it for its transparent larvae, as they can be used to observe the biological phenomena taking place inside in real time (using time-lapse microscopy*). It is, for instance, central to a project led by Sandrine Etienne-Manneville's team (Cell Polarity, Migration and Cancer Unit), which focuses on research into serious brain tumors, gliomas, the second most common type of cancer in children. "By injecting human glioma cells into the brains of the fish, we can study their behavior, particularly their migration, to shed light on the interactions between tumor cells and surrounding cells during brain tissue invasion" explains the researcher. "So, we hope to improve diagnosis of gliomas in patients by determining their invasive properties, and also to use this model to simply, quickly and physiologically test the effect of potential treatments for these tumors, by adding pharmacological compounds directly to the fish water."
*Cost of time-lapse microscope: €350,000.
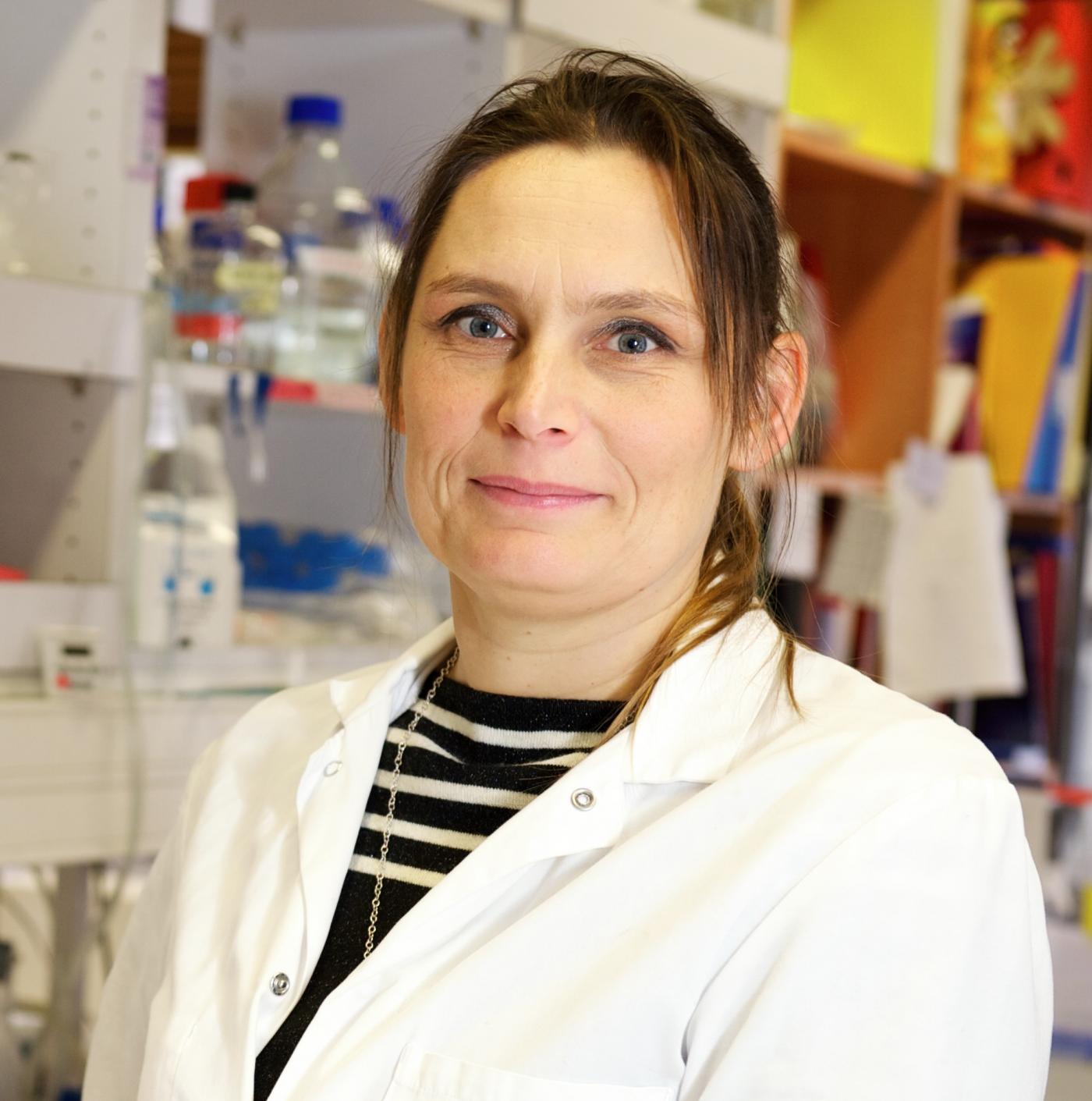
Sandrine Etienne-Manneville, researcher in the Cell Polarity, Migration and Cancer Unit, at the Institut Pasteur.
We hope to improve diagnosis of gliomas in patients by determining their invasive properties.
The nanoscopy era
To date, no fewer than seven Nobel Prizes in Chemistry have been awarded to scientists who have made advances in microscopy, including the 2017 prize presented to three researchers who developed cryo-electron microscopy (see Titan section below). Now better equipped to "see", researchers also have access to tools for interpreting their observations, for example automated image analysis using powerful algorithms (see below). Thanks to the phenomenal development of microscopy and related tools, researchers are today conducting studies they would never have imagined possible only a couple of years ago, i.e. monitoring the migration of new neurons in the brain or seeing cancer cells being destroyed by our immune cells (see below Graft rejection or cancer). Delving into the microscopic (and nanoscopic!) world unearths crucial information for fighting disease, speeding up, for example, the identification of key molecules, which are new targets for the drugs of tomorrow. As they make all this possible, microscopes could be classed as the "technological heroes" of biomedical research.
Image analysis – better than the human brain
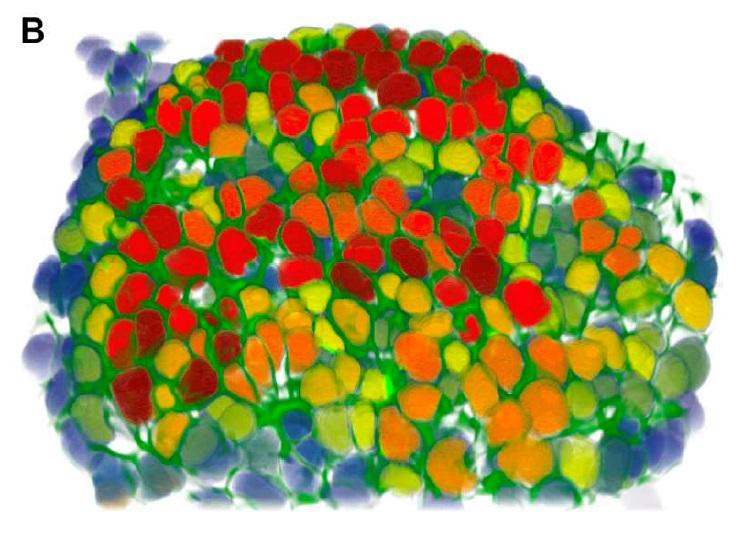
To help advance knowledge, it is not enough to have the best microscopes, you need to be able to analyze the images they generate. "Very often, you need to be able to count, for instance, how many hundreds of cells there are in the image with a particular shape?" explains Jean-Christophe Olivo-Marin, Head of the Quantitative Image Analysis Unit. "These tasks are laborious and not always reproducible. Depending on whether the experimenter performs them in the morning, afternoon or evening, the findings are not the same. As for the computer, it always produces the same result." Developed from applied mathematics of the 1980s, image analysis is central to cutting-edge technology today. "My team of mathematicians and physicists develops algorithms for biological imaging to be able to model, for example, how cells like ameba or metastatic breast cancer cells deform themselves to move about. Calculating the trajectory of a cell in an hour-long 3D time-lapse microscopy film would take a researcher days but it can now be done in real time thanks to image analysis. Our tools often exceed human capabilities."
Graft rejection or cancer – our defense cells seen in 3D and in real time!
A pioneer of imaging applied to immune system research, Philippe Bousso studies the behavior of our defense cells in pathological phenomena, such as graft rejection or cancer. The Dynamics of Immune Responses Unit that he directs at the Institut Pasteur produces very special films. For example, we can watch our natural defense cells give the "kiss of death" to cancer cells. "For this, we use a high-performance microscope* and appropriate laboratory-developed techniques for real-time in vivo observations. One of these techniques enables us to detect the death of a cell through a change in color. The ability to see cells in real conditions has revolutionized the notion we had of immune system function, as it is much more complicated than we thought. Its cells move about constantly, work together and create actual choreographies during a defense reaction."
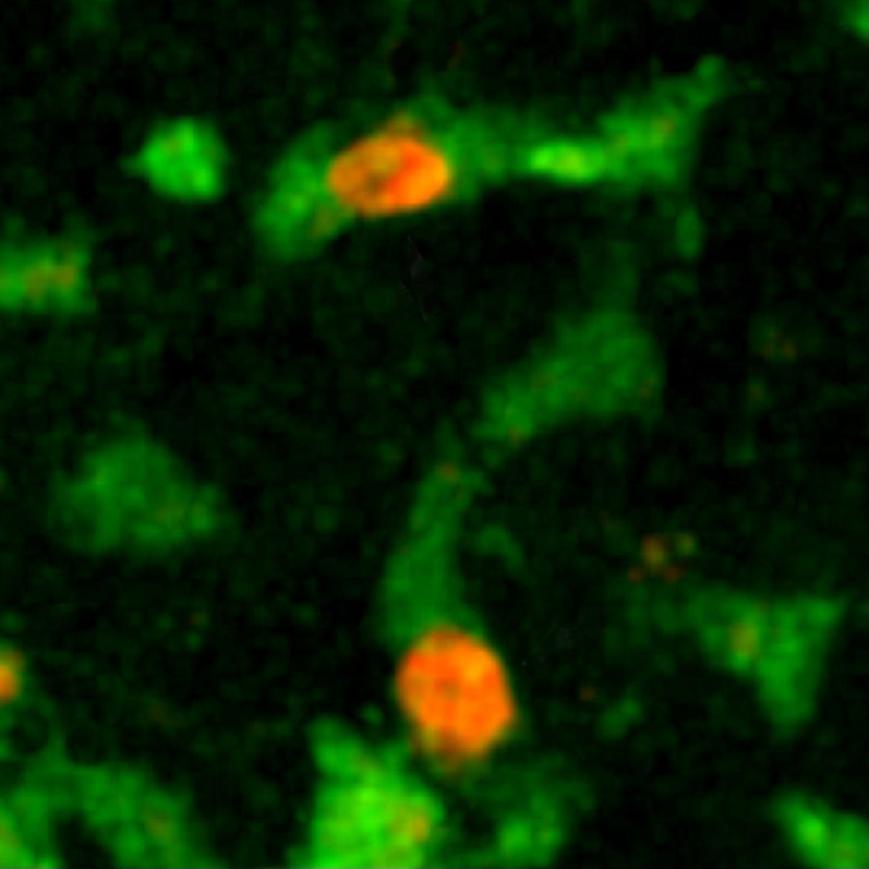
By observing the immune cell "ballet" during a skin graft rejection in 2011, the team was able to prove that a cellular mechanism was involved, paving the way for the development of new "anti-rejection" treatments. More recently, it has made significant progress in cancer immunotherapy (treatment that stimulates the patient's immune system so that it attacks its tumor cells), particularly by shedding light on the action of bone marrow transplants or molecules used to treat lymphoma (blood cancer). "Immunotherapy is rapidly expanding but often we know that it works without being able to explain why. Understanding how immune cells react during this therapy opens up new avenues for improving existing treatment or developing new therapeutic possibilities. In addition, dynamic imaging serves as a powerful tool for testing drugs. Their effect is seen instantly, in real time."
*A two-photon microscope. Cost: €400,000.
The Institut Pasteur is home to the world’s most powerful microscope...
Partly funded by generous donors, the world’s most powerful microscope, Titan Krios™, is currently being installed on the Institut Pasteur campus.
This ultra-high-resolution "cryo-electron microscope*" sits in a 3.8-meter high cabinet, connected to computer screens that reproduce the images. Its main advantage (but by no means the only one) is the resolution that can be reached, which is measured in tenths of a nanometer, i.e. atomic level. A whole section of hidden cellular machinery can be seen, as can the structure of proteins implicated in diseases, something which is useful for developing drugs. Titan Krios™ opens up new avenues for previously unimaginable research for scientists from many fields working on diseases as disparate as Alzheimer's and malaria. Another advantage comes from its ability to rapidly generate volumes of data. As it is automated, it can be used to observe up to 12 samples at the same time and collect images over several days. This results in considerable time savings for research.
Titan KriosTM gives the Institut Pasteur a closer look at life (video in French). Copyright: Institut Pasteur.
But such a technological marvel cannot be installed just anywhere. "It is so sensitive that a whole experiment could be ruined by someone raising their voice", joked one of its users in the UK. A building, featuring electromagnetic shielding and designed to dampen the slightest vibration, has been specially built to house it.
"A great many processes linked to pathologies studied at the Institut Pasteur are candidates for Titan Krios™ use and there is a long list of pending projects, which must go before a selection committee", explains Michael Nilges, Director of the Structural Biology and Chemistry Department. "We have also just set up a new research unit specialized in high-resolution electron microscopy, to make it easier to use."
*Samples are frozen at -180°C to fix the molecules in their environment.
Time-laps video of the installation of Titan KriosTM (video - subtitles in French). Copyright: Institut Pasteur.
…and imaging experts
There are many other microscopes on the Institut Pasteur campus, installed in research laboratories or, in the case of the most sophisticated ones, in two units at the Center for Innovation and Technological Research. One is dedicated to optical microscopy, the other to electron microscopy. "We help researchers choose the best method for their requirements and use one of our five high-resolution electron microscopes", explains Jacomine Krijnse-Locker, Head of the Ultrastructural BioImaging Unit. "For instance, they have been used to view aggregates formed by the chikungunya virus in mosquito salivary glands, Shigella entry into human cells, or the modification of the wall of bacteria responsible for meningitis under the effect of an antibiotic. In 2016, we helped with 85 research projects." Close by stands the Photonic BioImaging Unit and its 30 high-level optical microscopes, which are useful for observing living elements. For its director Spencer Shorte "the two microscopy units complement each other and work together on many projects. In addition to the service we provide, we carry out technological research to improve optical imaging methods, particularly applied to cell biology and microbiology. We want to push current boundaries even further!"
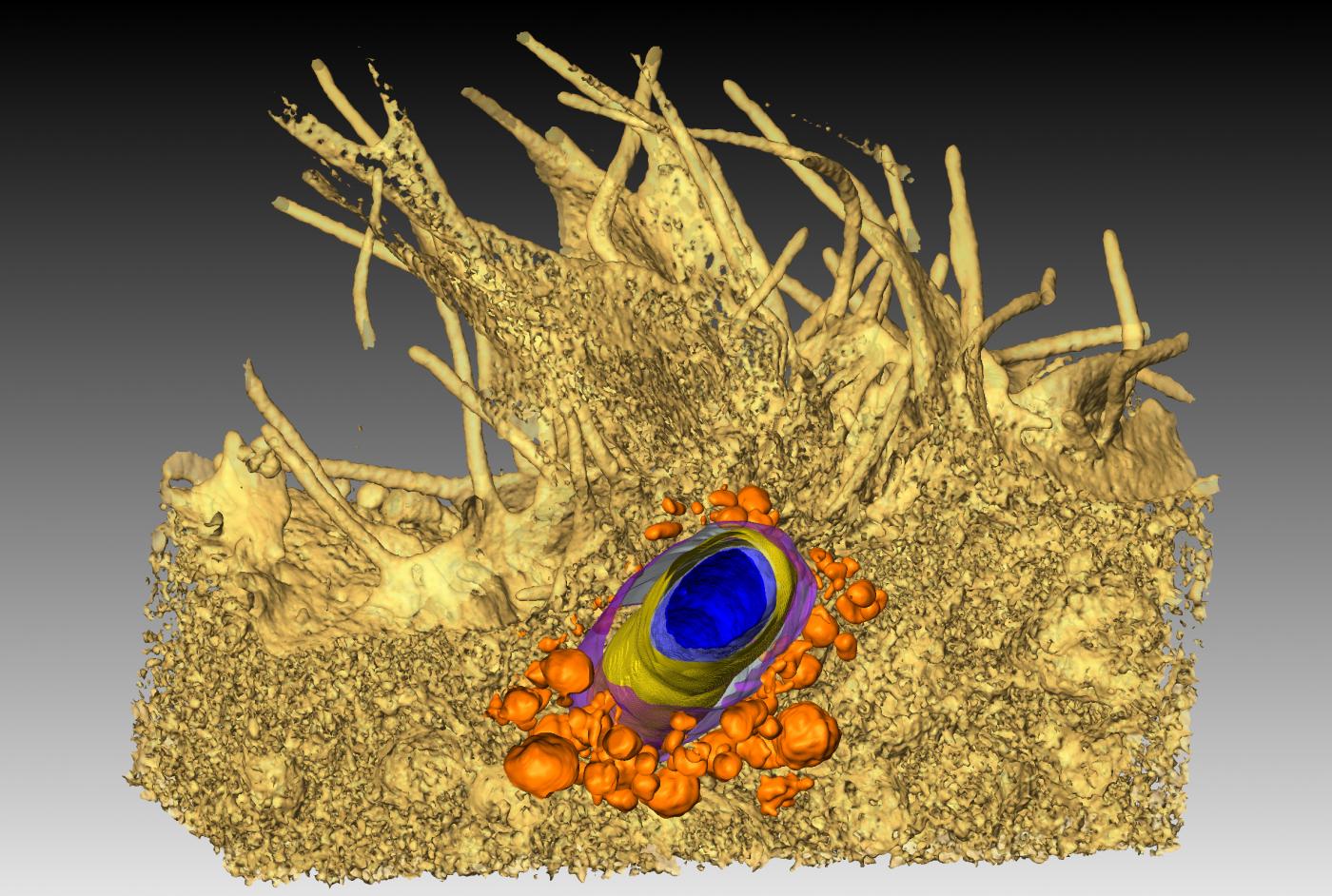
*Cost: between €700,000 and over €1m.