New weapons to fight bacteria
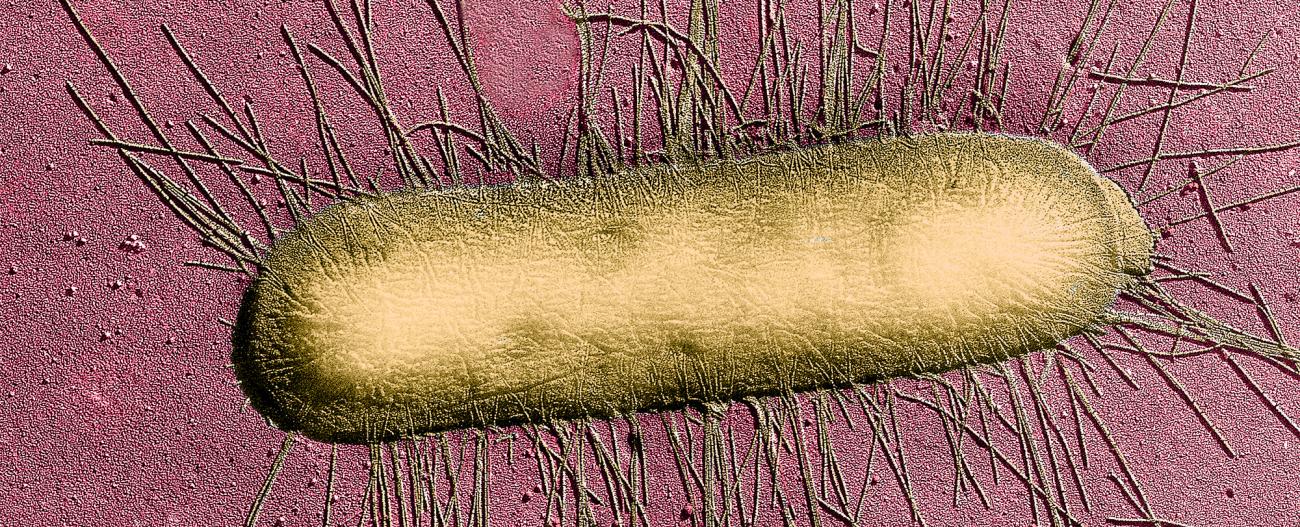
Pneumonia, bronchitis, ear infections, meningitis, tonsillitis, urinary tract infections, whitlow, septicemia, etc. Many infections are caused by bacteria. Bacterial infections have affected us all, and will continue to do so. Our doctors respond by treating such infections with antibiotics. These standard "weapons" against bacteria have saved millions of lives, and will save millions more. But abusive use in humans and animals has led to the accelerated emergence of bacteria that are resistant to these drugs. The efficacy of antibiotics is now seriously under threat. It has become crucially urgent to find new antibiotics, or to develop alternatives for fighting pathogenic bacteria.
Infections that were under control for a while are once more becoming serious
"One day no antibiotics may be left to treat common bacterial infections," warns the World Health Organization. Already, each year in Europe, 400,000 people – 158,000 of them in France – are infected by bacteria that are multi-resistant to antibiotics. When such bacteria are involved, an infection easily treated just a few years ago, and therefore considered "mild", may now kill a person. 12,500 deaths are attributed to this cause each year in France. Doctors are increasingly finding themselves in a therapeutic impasse with some patients, with no antibiotics having the slightest impact on their infection.
The figures speak for themselves: pneumococcal resistance to penicillin G was almost non-existent twenty years ago; in France, 27% of strains of this bacterium are now resistant; in 1 in 5 cases, hospital-acquired Staphylococcus aureus infections (nosocomial infections) are resistant to the preferred antibiotic. Over the last 10 years, the increasing antibiotic resistance of another bacterium, Escherichia coli, has also been a cause for concern: it may cause urinary tract infections – formerly described as mild – that many patients have trouble getting rid of.
The search for new antibiotics is a major challenge
The French Institute for Public Health Surveillance (InVS) made the following statement in November, concerning antibiotic consumption in the community (outside of hospitals): "France is one of the highest consumers of antibiotics in Europe, just behind Greece. It consumes 30% more than the European average, and three times more than the most prudent countries." Drug pressure is accelerating the emergence of resistant bacteria. The health authorities are therefore trying to reduce consumption in order to contain the increase in antimicrobial resistance. Hygiene measures in hospitals have been significantly stepped up over the last few years, in an attempt to slow the dissemination of these bacteria and resulting patient infection.
Although these are essential initiatives, they can only limit the problem. The presence of multi-resistant bacteria is a fact. One of the major challenges in biomedical research is finding new therapeutic weapons for fighting them. A number of avenues are being explored in research laboratories, including screening for new antibiotics, nanodrug design, therapy using "bacterial viruses", and the development of "smart antibacterials", to name but a few.
In addition, even if pathogenic bacteria are individually susceptible to antibiotics, they are able to avoid antibiotic action when grouped together in a community: they may form biofilms on the surface of some tissues in the organism and also on medical equipment such as catheters or implantable devices. The current antibiotic arsenal is unable to destroy biofilms, and research is under way to find dedicated solutions.
The following texts contain four examples of research into new antibacterials – four very different lines of attack against pathogenic bacteria but a single objective: to provide medicine with new weapons to fight bacterial infections.
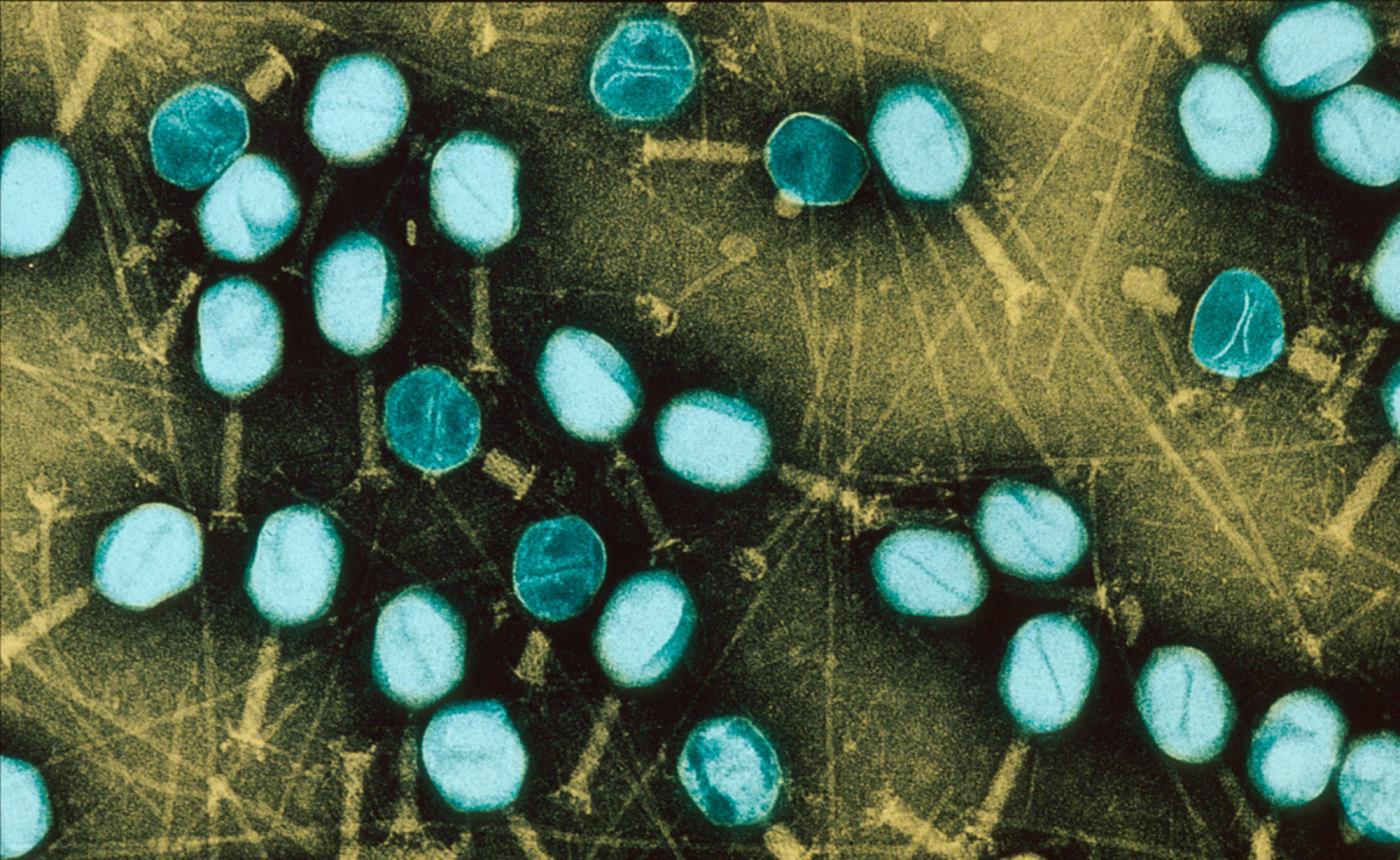
Although research into new antibiotics is essential in the face of growing antimicrobial resistance, other initiatives are examining the possibility of administering antibiotics differently – for example coating them in nanoparticles, an assembly of lipids or sugars. This formulation would have the advantage of administering antibiotics in smaller doses, which would slow down the emergence of resistance as well as enable the use of molecules that are currently no longer in use because they are poorly tolerated. The NAREB (Nanotherapeutics for Antibiotic Resistant Emergent Bacterial pathogens) project, launched in February 2014 and coordinated by the Institut Pasteur, unites 15 laboratories over 8 European countries around a single objective: the development of nanodrugs against two types of infection that are giving great cause for concern – methicillin-resistant Staphylococcus aureus (MRSA) and multi-resistant tuberculosis. MRSA causes a large number of serious nosocomial infections: affected people are 64% more likely to die than people infected with a non-resistant form of S. aureus. In 2014 nearly 480,000 people developed multi-resistant tuberculosis, with almost one-tenth of cases being "ultra-resistant" infections, reported in one hundred countries and against which even second-line antibiotic treatment is ineffective.
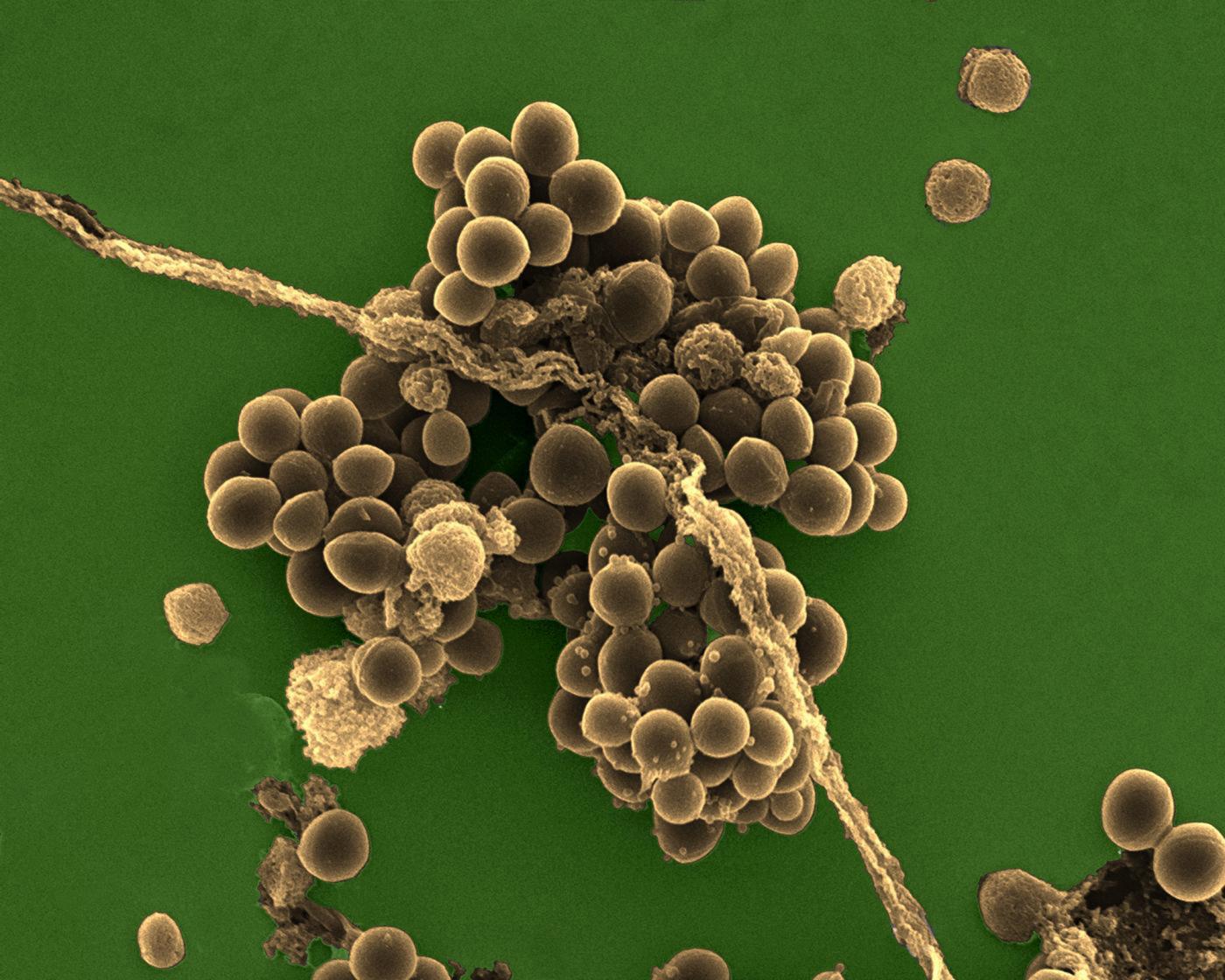
The tuberculosis crisis
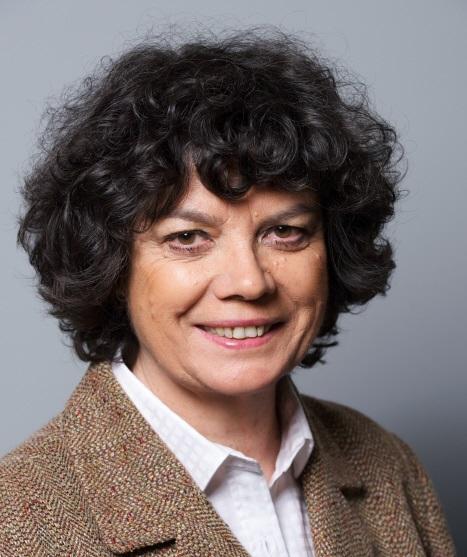
Pr Brigitte Gicquel, coordinator of the European NAREB project and head the Mycobacterial Genetics Unit at the Institut Pasteur.
The situation has been rendered even more critical, concerning tuberculosis, given that bedaquiline, the only new molecule for treating serious cases of multi-resistant tuberculosis, has shown a level of toxicity far greater than expected. The advent of new drugs and innovative formulations is therefore a more urgent requirement than ever.
Faced with disengagement by most of the industry, we in academic laboratories must work hard to find new antibiotics with the pharmaceutical companies that are still involved," added Professor Gicquel, who is a tuberculosis specialist and who, in parallel with her involvement in the NAREB project, is taking part in a number of international programs. Her laboratory is working on research into new antibiotics for fighting the tuberculosis bacillus using molecular screening methods, in collaboration with Hélène Munier-Lehmann (Chemistry and Biocatalysis Unit, Institut Pasteur) and the Institut Pasteur of Shanghai.
This is crucial research in the face of a disease that is the most deadly infection caused by a single pathogenic agent (ahead of AIDS and malaria): 9.6 million people, including over a million children, developed tuberculosis in 2014 and 1.5 million of them died, according to the World Health Organization. Currently, 3.7% of new tuberculosis cases worldwide and 20% of existing cases concern multi-resistant forms, complicating a care burden that is already onerous for drug-susceptible tuberculosis (which involves several months’ treatment with antibiotics).
Taking advantage of natural bacterial enemies
Scientists are increasingly focusing modern research methods on a therapy identified at the beginning of the 20th century, before the first antibiotics were discovered. It consists of attacking and destroying bacteria using their natural enemies – "bacterial viruses", known as bacteriophages, each of which is specific to a given bacterium and therefore infects only the host bacterium without destroying, for example, microbial flora.
Félix d’Hérelle gave the first description of the phenomenon of bacterial destruction by bacteriophages at the Institut Pasteur in 1917, and reported on the first experimental trials of "phage therapy". Two years later this new therapy was applied to humans, at a time when there was no weapon for fighting the bacteria responsible for causing many infectious diseases and countless deaths. Bacteriophage solutions were made commercially available, and phage therapy experienced a golden age until antibiotics arrived on the medical scene at the beginning of the 1940s and offered chemical molecules that were easily standardized industrially. Only some Eastern European countries have continued to use them into the present day. But current clinical trial standards have moved on a long way since then, and the therapeutic efficacy of these "healing viruses" therefore needs to be reassessed.
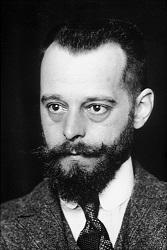
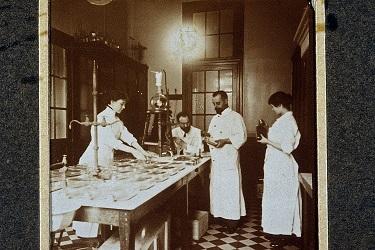
An alternative therapy for clinical evaluation
At the Institut Pasteur, Laurent Debarbieux’s group in the Department of Microbiology has been working on bacteriophages for several years, and has recently demonstrated their efficacy in the laboratory against two types of pulmonary infection. "We are primarily seeking to demonstrate the therapeutic potential of bacteriophages in the treatment of infections caused by antibiotic resistant bacteria," he explained. Part of this work is focused on the bacterium Pseudomonas aeruginosa, which is responsible for the pulmonary infections that often affect cystic fibrosis sufferers and are a major cause of death in these patients. One of the challenges was to confirm the ability of bacteriophages to act through the thick mucus that invades the respiratory system of patients. "In experimental models in the laboratory we proved that a cocktail of 10 bacteriophages has the ability to infect strains of P. aeruginosa present in the pulmonary secretions of patients," explained Laurent Debarbieux. "We are currently discussing the implementation of a clinical trial, in conjunction with a patients’ and clinicians’ association*."
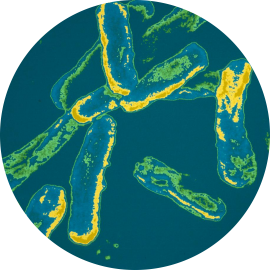
Another avenue of research consists of targeting strains of E. coli – a bacterium with dramatically increasing antimicrobial resistance, which is known to cause pneumonia in intensive-care patients. The team showed in the laboratory that one application of a single type of bacteriophage was as effective as treatment with standard antibiotics.
"We now need clinical trials to evaluate these therapeutic approaches," stressed Laurent Debarbieux. "A European trial is in progress to test phage therapy in E. coli and P. aeuginosa infections in severe burn patients. Our results now justify the launch of other clinical trials, despite regulations surrounding bacteriophages that are more complex than those relating to inert substances."
*The team is collaborating with clinicians at Necker, Bichat and Louis Mourier hospitals in Paris and the University Hospital in Montpellier
Besides the problem of antimicrobial resistance, antibiotics have a major drawback: they indiscriminately attack both good and bad bacteria. In particular, it is known that they destroy part of our microbiota, which is fundamental to the proper functioning of the human body. Recent advances in biotechnology have allowed the design of completely new weapons to target only harmful bacteria.
Biological "robots"
For this, scientists are developing an alternative therapy to antibiotics, known as phage therapy, using bacteriophages, or "bacterial viruses". But these bacteriophages cannot be administered in their natural state. Using genetic engineering, scientists are able to transform them into little biological robots for killing specific bacteria. This is how it works: only the bacteriophage envelope – the capsid – is used; its advantage is that it can penetrate bacteria, but not human cells. This capsid will therefore serve to deliver a "genetic circuit" to harmful bacteria: it comprises molecular scissors combined with a "guide", that will exclusively target a small, specific piece of DNA from the target bacterium within a resistance gene or virulence gene. The bacteriophage is thus converted into a Trojan horse: it injects the circuit into the bacterium and the genetic program is set in motion. The scissors "scan" the DNA. If they find a fragment of gene, they cut it. The bacterium dies. If they do not find a fragment of gene, there is nothing to cut. The bacterium survives.
Find David Bikard, head of the Synthetic Biology group at the Institut Pasteur, who explains smart antibiotics to eliminate bacteria in a targeted way, on the Youtube channel of the Institut Pasteur.
Scientists have dubbed these new antibacterial tools "eligobiotics", since the Latin eligo means to choose or select. "Since each bacteriophage is specific to a given bacterium, we must manufacture eligobiotics using different capsids, depending on which bacteria are being targeted," explained David Bikard, who heads up the Synthetic Biology Group at the Institut Pasteur. "Eligobiotics have given us the first opportunity to destroy bacteria in such a targeted way," he added. "However, they are not intended to replace antibiotics. They will never be as effective and as fast-acting as a conventional antibiotic molecule. But they could be very useful in people infected by bacteria that are multi-resistant to antibiotics. Other uses are also possible. For example, we know that microbiota imbalances are the root cause of many diseases. Eligobiotics could enable us to restore the balance by destroying harmful bacteria in the microbiota."
The team is collaborating with clinicians at Necker, Bichat and Louis Mourier hospitals in Paris and the University Hospital in Montpellier.
Acne and Crohn’s disease
David Bikard is also Chief Scientific Officer of the start-up company Eligo Bioscience, which took root at the Institut Pasteur and aims to develop eligobiotic treatments, in the first instance against acne and Crohn’s disease. In the case of acne, current antibiotics do not discriminate between useful P. acnes bacteria and those that cause the skin disease, and serious side effects are associated with their use. Crohn’s disease could in part be linked to an imbalance in the intestinal microbiota. In both cases, the aim is to target certain bacterial populations very specifically.
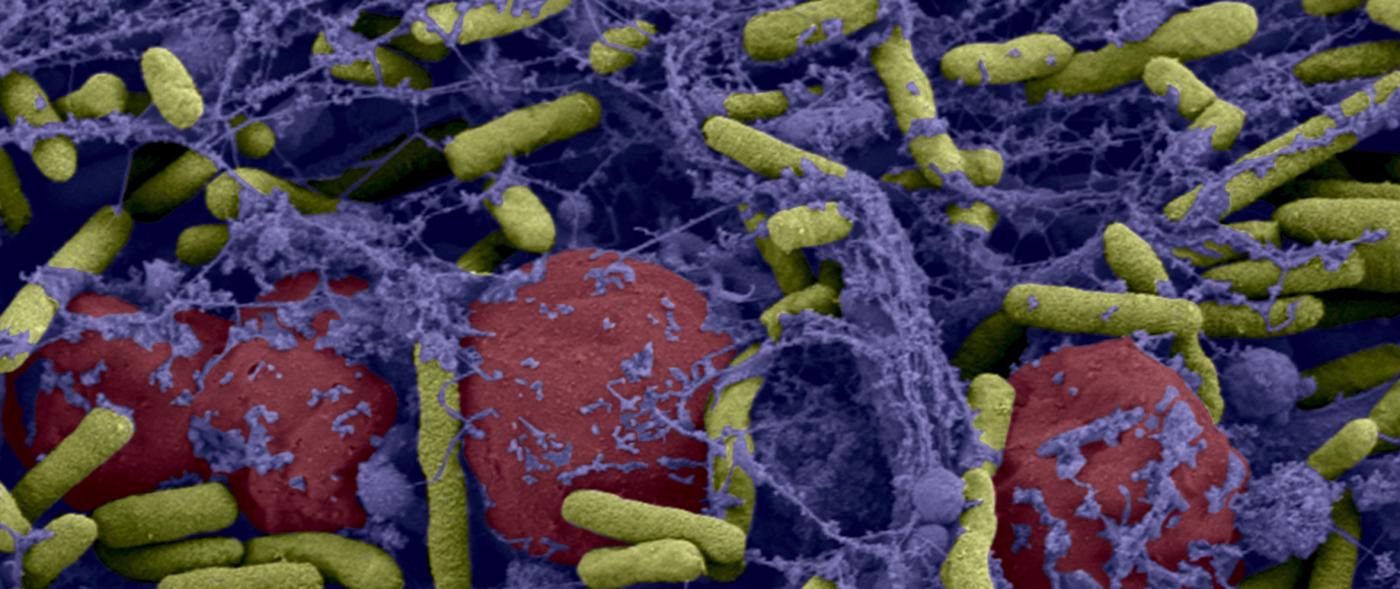
Bacteria are even more difficult to eradicate when they live in a community. On our tissue, on catheters and medical devices, bacteria may form "biofilms": these films adhere to the surface, secrete a coating – the matrix –, multiply and group together to form a mass that may contain millions of billions of bacteria. Since there is strength in numbers, these bacterial biofilms become tolerant to antibiotics: even if, individually, they are susceptible to antibiotics, the latter no longer have an effect when the bacteria are organized into a biofilm (and the problem is, of course, exacerbated if the biofilm comprises resistant bacteria). Medicine is currently being handicapped by these bacterial communities, and the fight against biofilms has become a significant challenge for biomedical research. This is particularly relevant when we know that biofilms are involved in 60% of hospital-acquired infections (nosocomial infections).
Photo above: Biofilm of bacteria Pseudomonas aeruginosa formed in a central catheter. In yellow Pseudomonas aeruginosa and red blood cells. © Institut Pasteur / A. Chauhan, JM Ghigo, C. Beloin. Photo by Cellular Biology Laboratory - Electron Microscopy, Faculty of Medicine of Tours. Colorization JM Panaud.
From implantable ports to medical devices
Jean-Marc Ghigo’s team at the Institut Pasteur (Genetics of Biofilms Unit) specializes in the study of biofilms, analyzing their formation and the various aspects of bacterial community life. He described the mechanism that stops antibiotics being effective: "If an antibiotic acts on a biofilm many bacteria will be killed, but some, known as "persistent", will survive the treatment, and the biofilm will reform." Fortunately, solutions are in sight. In collaboration with clinicians*, the Institut Pasteur team conducted a study on implantable ports, which are small tubes implanted under the skin that allow the administration of drugs, particularly in the context of chemotherapy treatment for cancer. "These ports are infected with biofilms in 4 to 5% of cases," explained Jean-Marc Ghigo. "When an infection is suspected, the port must be removed, which is not a minor procedure, particularly in immunocompromised patients." Today, between two uses of a port, an antibiotic "lock" is applied to limit the formation of biofilms; this consists of injecting a small volume of antibiotics into the port. "We are looking to develop a "curative" lock that is able to eliminate any biofilms in implantable ports. To do this, we combine a conventional antibiotic with a molecule that attacks the biofilm matrix while increasing the penetration of the antibiotic into persistent bacteria. We have proven the efficacy of this combination in the laboratory." This new antibacterial "lock" is currently being manufactured by Hospices Civils de Lyon with a view to future clinical trials. Another avenue of research is being studied in the unit, involving the possible prevention of biofilm formation. "A molecule is "grafted" onto surfaces, making them "slippery" so that bacteria are unable to attach themselves or adhere to these surfaces," explained Christophe Beloin, a scientist who is conducting these experiments with Jean-Marc Ghigo. "For the moment, long-term results with this strategy are proving difficult, but it could be used over the short term, when fitting a medical device, for example."
*The Genetics of Biofilms Unit is collaborating with David Lebeaux of the Necker and George Pompidou hospitals in Paris, and with clinicians at Dupuytren hospital in Limoges.
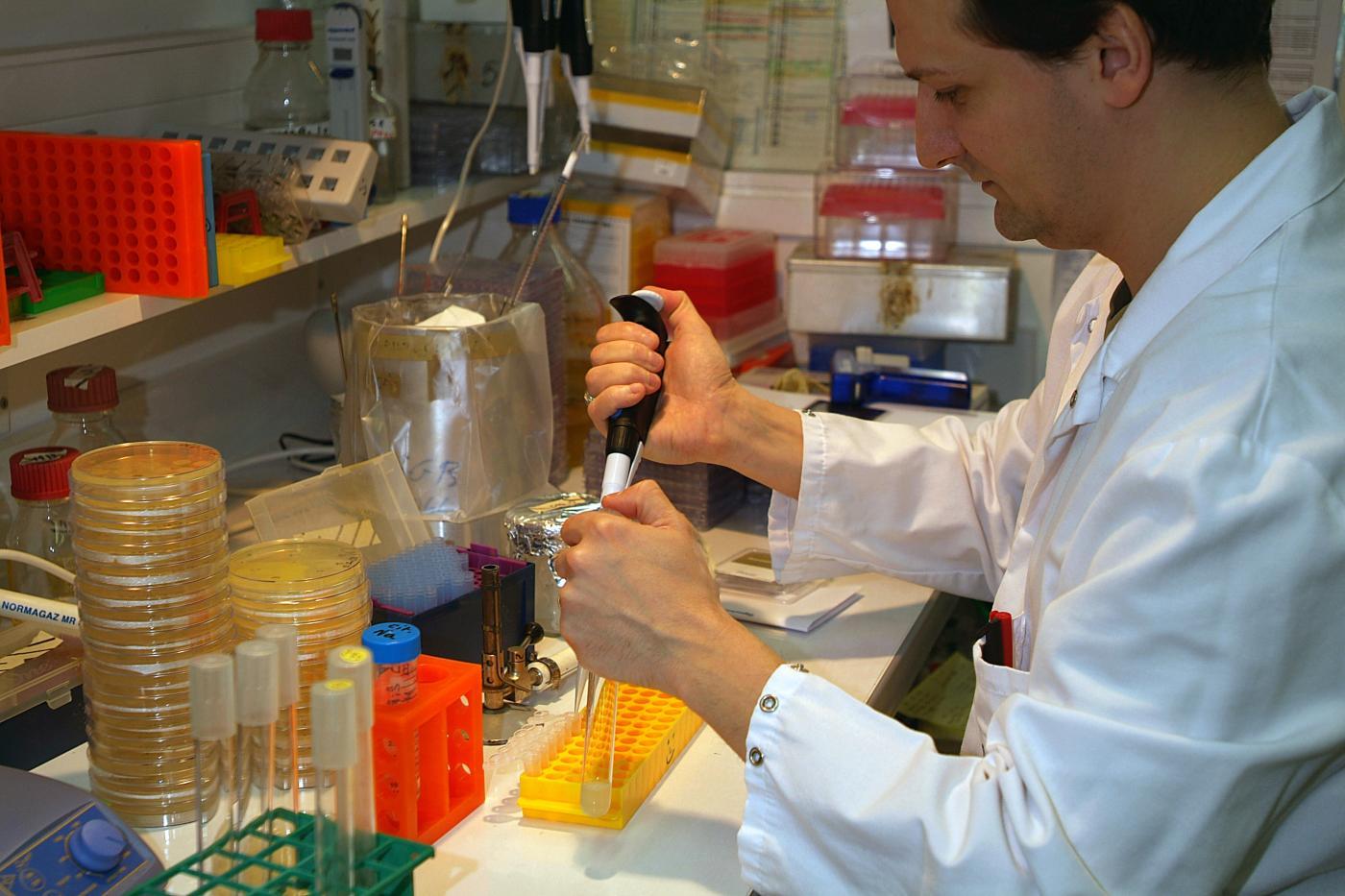