Resistance, resilience. In an ever-changing world, adaptability is crucial
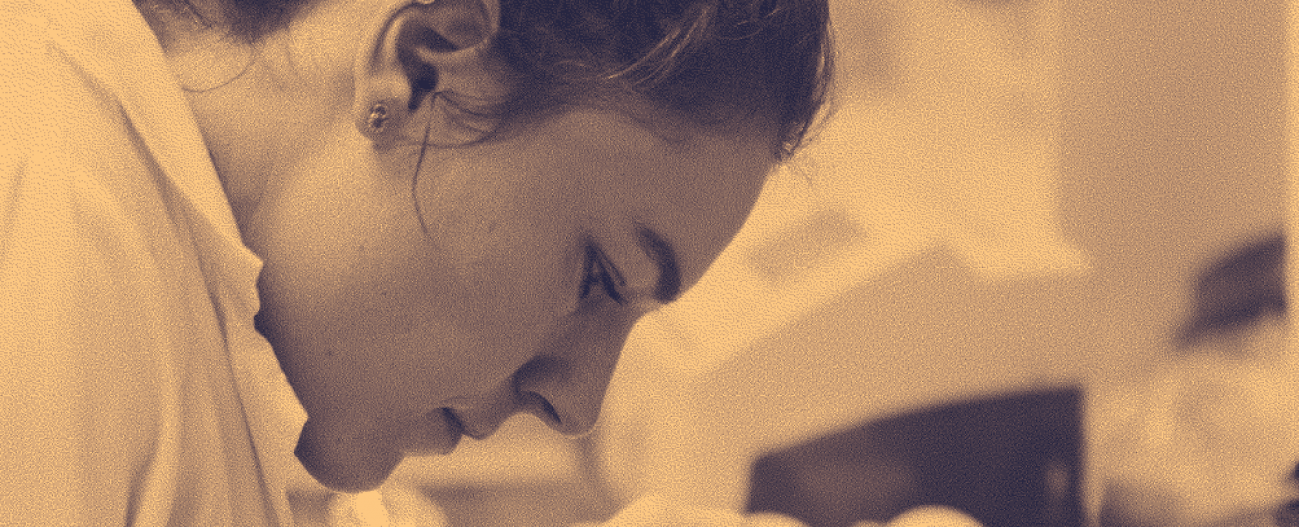
Adaptation is a universal phenomenon. It occurs whenever anything exceeds its natural limit – examples include microbial resistance, scientific innovation and the evolution of the human immune system. This special feature highlights the incredible complexity of adaptation processes.
Adaptation: the secret to survival
With Erik Orsenna, French novelist, member of the Académie Française and Ambassador of the Institut Pasteur
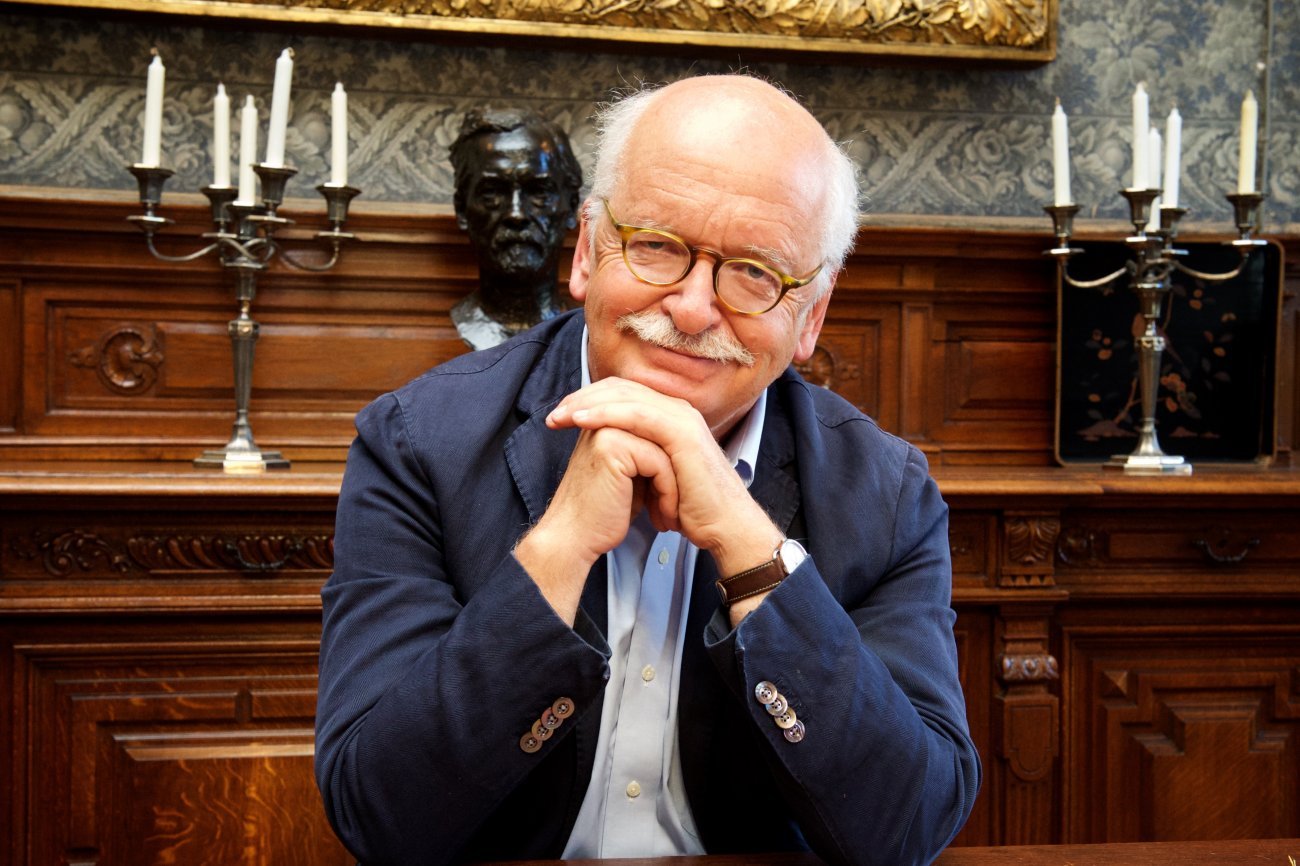
As vertebrates, we are vastly outnumbered by insects, of which there are 8 million species compared to our 8,000 …Why are they so successful? Every time I have a question about the living world, I ask Professor Gilles Bœuf*.
In my view, there are six reasons for insects' success, which can be summed up by the following equation: Small + eat anything + live anywhere + reproduce intensively + like to live in communities + diversity = skilled adapters.
That's the secret to survival: adaptation!
Insects aren't the only creatures that use this kind of agility to survive. All living beings and populations are constantly adapting. This of course also applies to those peskiest of lifeforms: pathogens.
Words are also constantly adopting disguises, pairing up and evolving. In fact the reason that languages adapt from generation to generation is that they too are living things!
*Editor's note: a biologist and former president of the French Museum of Natural History in Paris
What is adaptation?
With Simonetta Gribaldo, Head of the Evolutionary Biology of the Microbial Cell Unit
Stage 1
Adaptation, noun: the process of changing to suit different conditions (Cambridge Dictionary). Adaptation is a reaction, a necessary development. We adapt to adjust to a situation or to our environment.
Stage 2
For living beings, adaptation is also a necessary biological process, a beneficial adjustment, a way of fully making the most of one's environment, of getting the best out of it. Adaptation reflects an ability to reinvent oneself. It is an iterative process that permanently challenges the status quo in an ever-changing world. It is a reaction by individuals to everything that surrounds and shapes them – and is shaped by them in turn.
Scientists are no exception to this rule – they also regularly demonstrate adaptability. The scientific approach is one of constant adaptation: we adapt our knowledge, our theories, our processes, our view of the world. Adaptation forces us to face up to the fleeting nature of things and prompts us to respond with agility, humility and invention. For while it is necessary and inevitable, adaptation is also a driving force, underpinned by the interdependence that characterizes our existence.
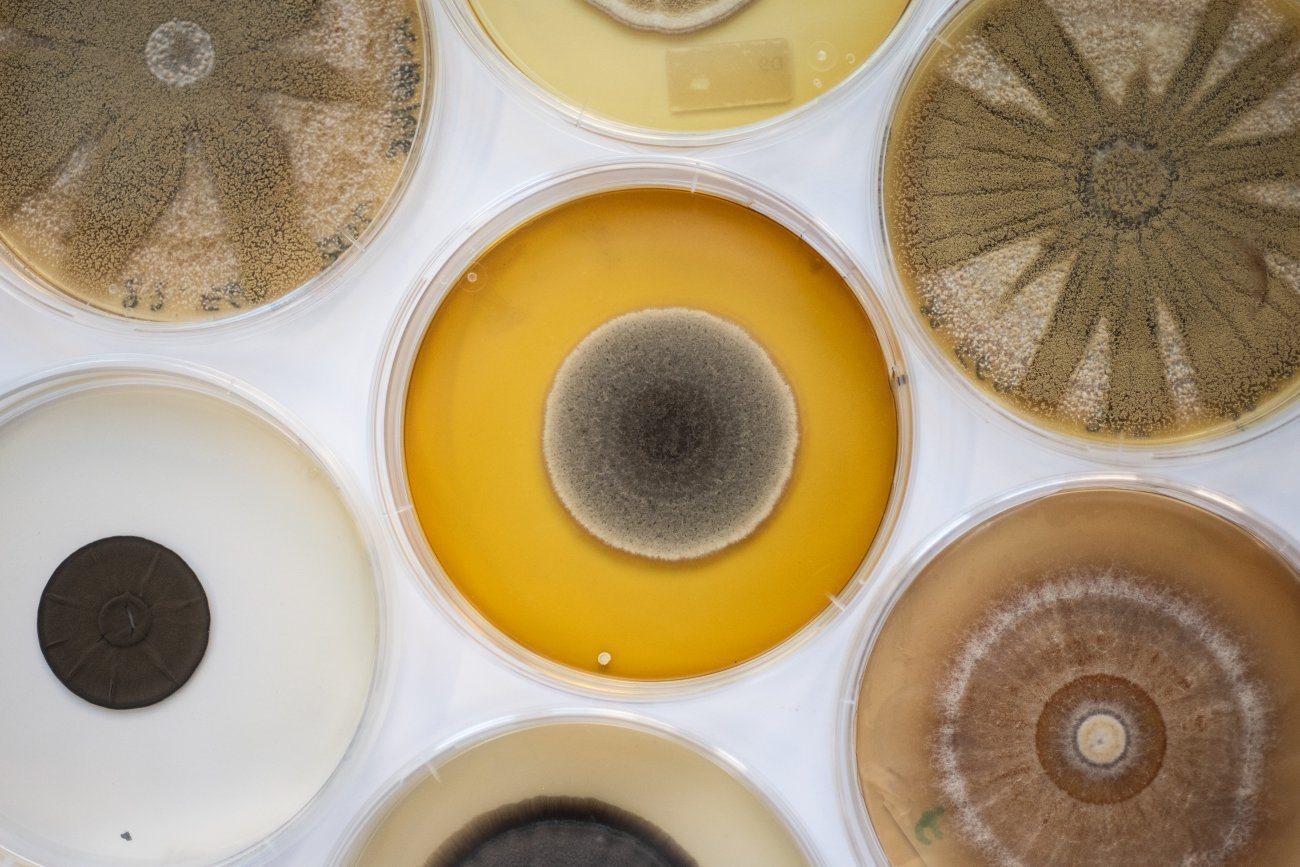
Petri dishes with cultures of different fungi - Credits : Institut Pasteur - François Gardy
A tale of resistance
With Paola B. Arimondo, Head of the Epigenetic Chemical Biology Unit, and Philippe Glaser, Head of the Ecology and Evolution of Antibiotic Resistance Unit.
Microbes and antimicrobials: a never-ending battle
Antimicrobial resistance is growing at a dramatic rate and raising concern in every corner of the planet. In 2019 it was included in WHO's list of the ten greatest threats to global public health. Antibiotic-resistant bacteria alone were responsible for 1.3 million deaths in 2019. That same year, the Institut Pasteur made antimicrobial resistance one of the three priorities of its Strategic Plan. Although antibiotic resistance grabs all the headlines, the problem is much broader: every category of drugs used to prevent and treat infections in humans, animals and even plants – including antivirals, antifungals and antiparasitics – is facing growing resistance.
While the situation is alarming, it is not surprising: it is only natural that microorganisms will evolve over time to thwart strategies aimed at eradicating them. But antimicrobial resistance is rendering treatments ineffective and jeopardizing our ability to contain infections over which we had temporarily gained the upper hand. Resistance has wide-ranging consequences: it drives epidemic spread, opens the way to a resurgence in "forgotten" infectious diseases, complicates treatment for patients and leads to soaring costs for health systems.
In Sub-Saharan Africa, 50% of HIV-positive infants are infected with a virus that is resistant to common antivirals. After South-East Asia and the Western Pacific, the Institut Pasteur has revealed that the malaria parasite is resisting state-of-the-art antimalarial drug artemisinin in Eritrea. Elsewhere, mosquitoes are evading insecticides, and fungi like Candida auris, a common problem in hospitals, are no longer responding to common antifungals. Whenever a new area of resistance develops, the scientific community tries to understand, prevent and innovate in a bid to come up with new strategies in this never-ending battle.
Why and how do bacteria become resistant?
Antibiotics represent one of the most important breakthroughs in modern medicine, starting with the discovery of penicillin by Sir Alexander Fleming in 1928. Antibiotics may be broad or narrow spectrum, and they are used to kill bacteria or stop them proliferating. But bacteria are like any living organism – when faced with a threat to their survival, they evolve. Just as natural selection enabled animals, including humans and their immune system, to adapt to their pathogenic environment, bacteria have developed resistance to our attempts to eradicate or at least contain them. This is what we now refer to as antimicrobial resistance.
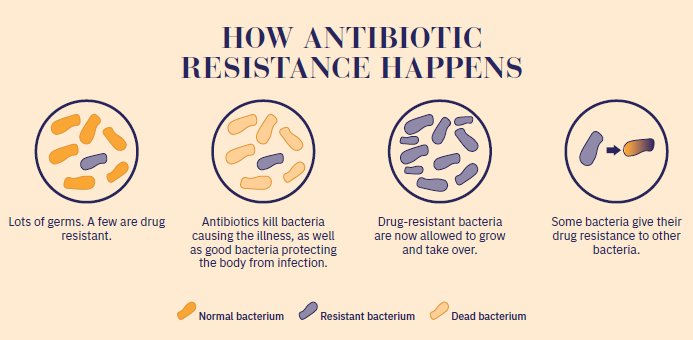
Bacteria can acquire resistance in two ways. The first is mutation. In this case, bacteria develop mutations that mean they are no longer susceptible to a given antibiotic, and natural selection enables these resistant bacteria to reproduce more effectively and become dominant. This is the only mechanism used by the tuberculosis agent Mycobacterium tuberculosis and the main mechanism employed by Pseudomonas aeruginosa, bacteria that infect the lungs of people with cystic fibrosis. Escherichia coli's resistance to fluoroquinolones is also essentially caused by mutations. Bacteria can also develop resistance by acquiring resistance genes. Bacteria exchange plasmids, a type of genetic material that confers resistance. Plasmids can be transferred between bacteria in different genera, and between pathogenic and non-pathogenic bacteria. This is the main mechanism by which Escherichia coli and Staphylococcus aureus became resistant to methicillin, for example.
Reservoirs of antibiotic resistance, frequently created by "friendly" commensal bacteria in our microbiota, are fueled by widespread use of antibiotics, especially indiscriminate broad-spectrum antibiotics. The more we eliminate non-resistant bacteria, the more we make room for those resistant bacteria that manage to survive. We are at risk of losing hard-won progress through dosage errors, wrongly using antibiotics to treat viral infections, stopping antibiotic treatment early and using out-of-date or fraudulent products. Antibiotics are a double-edged sword that need to be handled with caution and used in a targeted way.
Resistant bacteria are responsible for infections that are difficult or impossible to treat. Some are resistant to several antibiotics and are known as multidrug-resistant (MDR) bacteria. They can even be resistant to all existing antibiotics ("pan-resistant") and lead to a therapeutic impasse. The most alarming MDR bacteria include Enterobacteriaceae family members Escherichia coli and Klebsiella pneumoniae (bacteria in the digestive tract responsible for a large number of infections); Mycobacterium tuberculosis; and Staphylococcus aureus and Pseudomonas aeruginosa, both frequently responsible for hospital-acquired infections. Some resistant bacteria can cause large-scale epidemics, like in Yemen, which is dealing with the most severe cholera outbreak in modern history. The Institut Pasteur has identified the plasmid conferring multiple antibiotic resistance on Vibrio cholerae for this outbreak. Antimicrobial resistance also concerns resistance to antiparasitics, antivirals and antifungals, as mentioned above.
Public enemies
The seven bacteria responsible for the most cases of antibiotic resistance in hospitals :
- Enterococcus faecium, gut bacteria known to have developed resistance to vancomycin
- Staphylococcus aureus, normally present in the pulmonary tract and on the skin, increasingly resistant to methicillin and more rarely to vancomycin
- Klebsiella pneumoniae, naturally present in the soil and in the skin, mouth and gut microbiomes, has developed resistance to carbapenems
- Acinetobacter baumannii, responsible for a great number of hospital-acquired infections, resistant to multiple antibiotics including all β-lactam antibiotics
- Pseudomonas aeruginosa, lives in soil and in damp and extreme environments. Very hard to treat as resistant to multiple antiseptics and antibiotics
- Enterobacter spp., causes many urinary and lung infections but still sensitive to colistin and tigecycline
- Escherichia coli, gut bacteria that are often harmless and even beneficial, but some strains are pathogenic and multidrug resistant
Fighting back
Antimicrobial resistance is a worldwide phenomenon that is affecting all living species. In 2015, WHO launched its Global Antimicrobial Resistance and Use Surveillance System (GLASS), and several databases have been compiled to record every occurrence of antimicrobial resistance worldwide. In 2002, the European Union banned all growth-promoting antibiotics used in animal feed, a measure that reflects the "One Health" approach that has developed since the early 21st century, based on the idea that the health of humans, animals and the environment is interlinked. In France, a national antibiotic resistance strategy was adopted by the Ministry for Solidarity and Health in 2022. But although there is widespread awareness of the problem, there are huge disparities and discrepancies from one country to the next: the phenomenon of antimicrobial resistance is by no means the same everywhere. A multidisciplinary team from the Institut Pasteur has developed a statistical model to analyze the data collected since 2004 in 51 countries. Their conclusion was that there are multiple determinants of antimicrobial resistance, ranging from socio-economic factors (GDP per capita) and climatic aspects (exposure to extreme meteorological events, average temperature in the country) to the quality of the health system and the volume of antibiotics consumed. Antimicrobial resistance prevention strategies need to be adapted to the local context and the infectious agents targeted.
But despite the need for a targeted approach, there are several key objectives that systematically apply. The first is a need to better understand the emergence and spread of resistance by drawing on epidemiology, genomics, statistics, evolutionary biology, structural biology, chemical biology and modeling. This research can help calibrate and rationalize the use of existing antimicrobials. The second is to identify new drugs and therapeutic strategies in response to resistance, by synthesizing specialized chemical molecules, exploring new targets (such as elements involved in host-microbe interactions and the life cycles of infectious agents), carrying out phenotypic and molecular screening, and phage therapy4 (discovered at the Institut Pasteur in 1917). Prevention (vaccines, health measures, training and education), surveillance and diagnostic development are also key to tackling antimicrobial resistance. Every avenue needs to be explored. The use of artificial intelligence to process huge volumes of data and identify novel molecules and biomarkers is particularly promising. The final objective is to improve prevention by adopting guidelines on the use of antimicrobials worldwide, for all aspects of human and animal health. We also need to introduce more effective wastewater treatment measures and restrict human-animal interactions. The goal is clear: we have to do all we can to delay the arrival of a post-antibiotic era in which everyday infections once again become life-threatening.
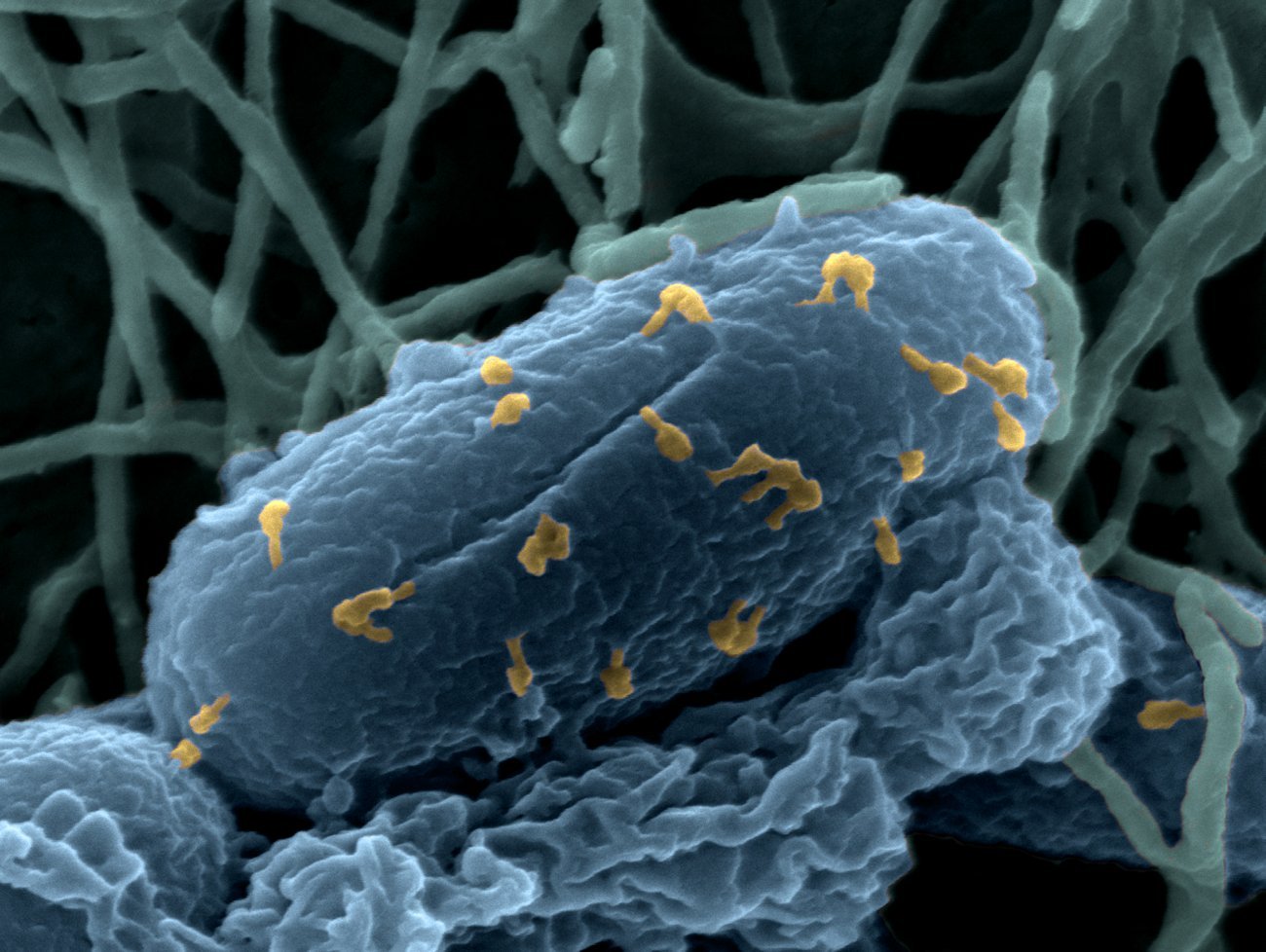
Bacteriophages on an Escherichia coli bacterium - Credits : Institut Pasteur/Chantal Le Bouguénec, Laurent Debarbieux, Perrine Bomme, Jean-Marc Panaud.
Weapons
Some of the research avenues to tackle antibiotic resistance at the Institut Pasteur
- Novel treatments and phage therapy
Scientists from the Institut Pasteur and the CNRS have demonstrated in vivo that bacteria are capable of regulating their gene expression to evade the many bacteriophages in the gut environment (Cell Host & Microbe, April 13, 2022). The same team has developed a mathematical model to predict the effectiveness of phage therapy in treating lung infections (Cell Reports, May 17, 2022).
- Understanding antibiotic resistance
Plasmids are DNA molecules outside the chromosome that can carry antibiotic resistance genes. They can move between bacteria via a process known as bacterial conjugation. Scientists in the Microbial Evolutionary Genomics Unit have revealed that some plasmids contain sequences enabling them to hijack proteins from other plasmids so they can be transferred from one bacterium to another – what we might call conjugation by hitchhiking. Their research means that we can now predict the transfer mechanisms in 90% of the known plasmids in bacteria (Nucleic Acids Research, November 29, 2022).
Another team, composed of scientists from the Genetics of Biofilms Unit and Rakuno Gakuen University in Hokkaido, Japan, has demonstrated that the biofilms formed by bacteria to survive in their environment encourage the emergence of antibiotic resistance (Communications Biology, March 16, 2023).
Mosquitos adept at adapting
With Anna-Bella Failloux, Head of the Arboviruses and Insect Vectors Unit
Mosquitoes transmit several epidemic diseases that are progressing an alarming rate. The aim of the vector control strategies employed to tackle them is always to try to keep one step ahead. But the adaptation and resistance developed by both mosquitoes in the genus Aedes and the pathogens they and other insects transmit represent a challenge for prevention, screening and treatment protocols. Two studies published by Institut Pasteur teams in 2023 shed light on these developments.
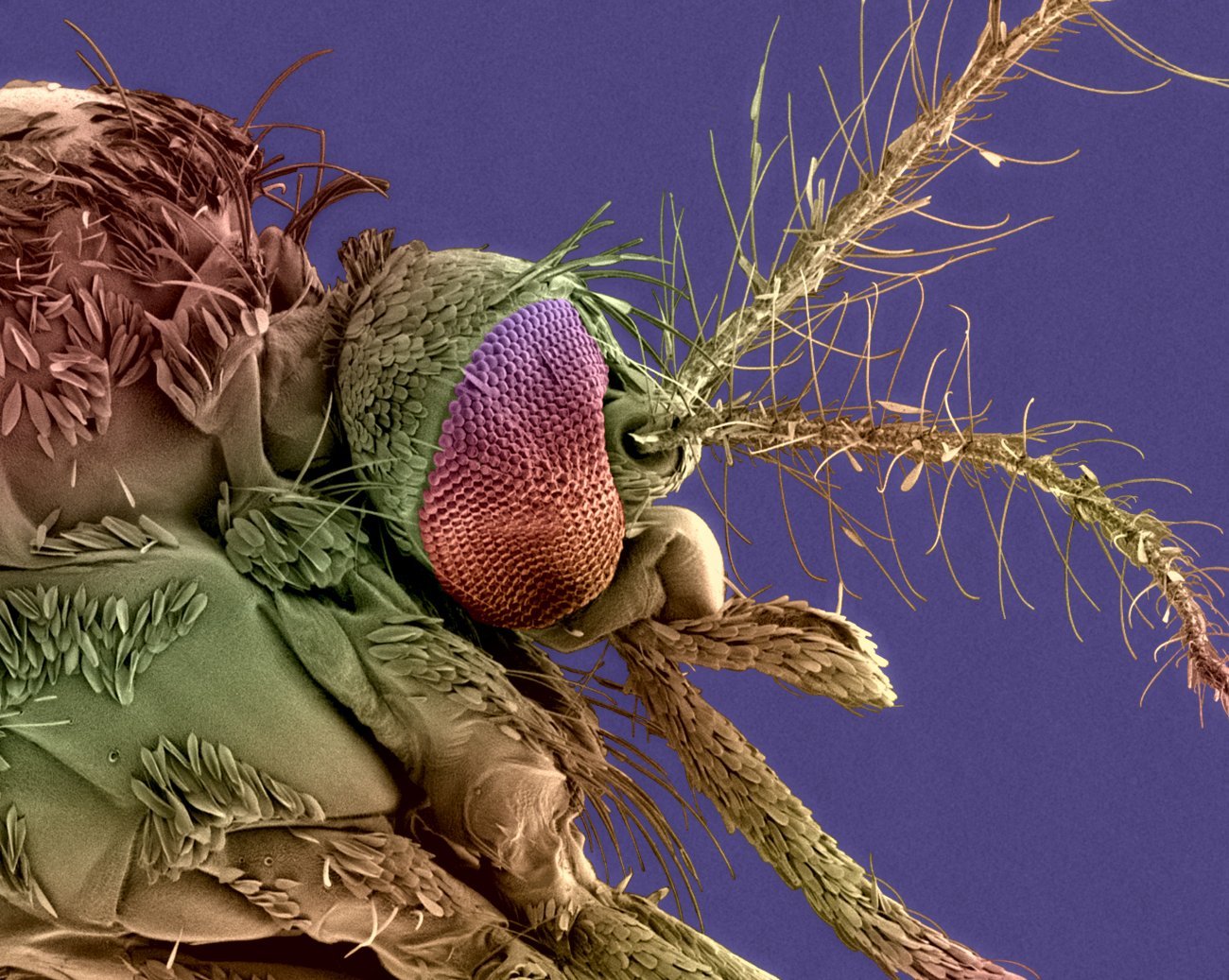
Head of a female Aedes albopictus mosquito, the vector for dengue and chikungunya - Credits : Institut Pasteur/Christine Schmitt, Plate-Forme Microscopie Ultrastructurale - Anubis Vega Rua, Laboratoire Arbovirus et Insectes Vecteurs - Colorisation Jean-Marc Panaud.
Expansion of the tiger mosquito to temperate regions
The tiger mosquito (Aedes albopictus) has come a long way from its native South-East Asia. Although originally a tropical species, it can now be found in large numbers in temperate regions from America to Europe, including mainland France, where it has already spread to four-fifths of the country. And Aedes albopictus has not come alone: it is one of two vectors of the chikungunya virus and is responsible for a number of indigenous cases reported far from endemic areas since 2007, including in Europe.
A team of scientists from the Institut Pasteur and Université Paris Cité focused on the impact of climate, especially temperature, on the transmission of pathogens by mosquitoes. Although the tiger mosquito is used to an average of 28°C, lower temperatures do not seem to prevent it from spreading the virus
The results of the study, published in the Journal of Travel Medicine, reveal an example of mutual adjustment between the virus and its vector in response to the environment. Temperature profoundly alters the gene expression and bacterial microbiome of mosquitoes and the genetic diversity of the chikungunya virus. But although lower temperatures result in different molecular processes, the mosquito is capable of transmitting the virus just as effectively at 20°C as at 28°C. This adaptation raises fears of a spread of chikungunya in the absence of vaccines and treatments.
Malaria under the radar in Eritrea
In recent years, Plasmodium falciparum, the parasite responsible for the most severe forms of malaria, has become increasingly resistant to rapid screening techniques and artemisinin-based therapy. A study coordinated by scientists from the Institut Pasteur has revealed a new focus of resistance in Eritrea, the most worrying to date because it shows that the parasites are capable of evading rapid tests and that their vectors are becoming resistant to insecticides. The conclusions of this research support the urgent introduction of novel vector control strategies in response to these adaptations.
Adaptation of our immune system
With Lluis Quintana-Murci, Head of the Human Evolutionary Genetics Unit
In the 1950s, the scientist J.B.S. Haldane theorized that the genetic mutation responsible for abnormalities in red blood cells observed in some African populations had persisted in those populations because it provided protection against malaria. The theory that pathogens are among the strongest selective pressures faced by humans has since been confirmed by several studies. But how does this selective pressure affect the immune system? And what are the consequences for the risk of developing inflammatory or autoimmune diseases? In a study led by a team from the Institut Pasteur, scientists analyzed genetic mutations in hundreds of individuals living in Europe over the past 10,000 years to reconstitute the evolution of the human immune system.
What is paleogenomics?
Paleogenomics is a relatively recent discipline that studies the DNA from fossil remains of humans, animals, plants or even microbes. It has already led to major discoveries about the history and evolution of humans and human diseases, and even resulted in the 2022 Nobel Prize in Medicine being awarded to Svante Pääbo for his sequencing of the Neanderthal genome. At the Institut Pasteur, paleogenomics has been used to reconstitute the history of tuberculosis and elucidate its impact on the human genome.
An age-old mystery finally solved
The scientists from the Institut Pasteur, Université Paris Cité, the CNRS and the Collège de France used paleogenomics to go back over 10,000 years of human history and try to trace the evolution of hundreds of thousands of genetic mutations over time. The study, published in the journal Cell Genomics, involved analyzing the genome variability of more than 2,800 individuals who lived in Europe over a period covering the Neolithic, the Bronze Age, the Iron Age, the Middle Ages and the present.
This detective work revealed a series of mutations that had occurred in response to "positive" natural selection, reducing the risk of developing infectious diseases. These mutations, which demonstrate a genetic adaptation to the pathogenic environment, began relatively recently in the grand scheme of human history, in the Bronze Age, around 4,500 years ago. The "sudden" acceleration in adaptation can be explained by the population growth during this period and the spread of infectious diseases as a result of increasing contacts between populations. At the same time, the scientists observed that mutations associated with a higher risk of inflammatory disorders, including Crohn's disease, have become more frequent over the past 10,000 years.
"These results suggest that the two phenomena are linked: the risk of inflammatory or autoimmune disorders has increased, at least in large part, because of a positive selection of mutations improving resistance to infectious diseases," explains Lluis Quintana-Murci.
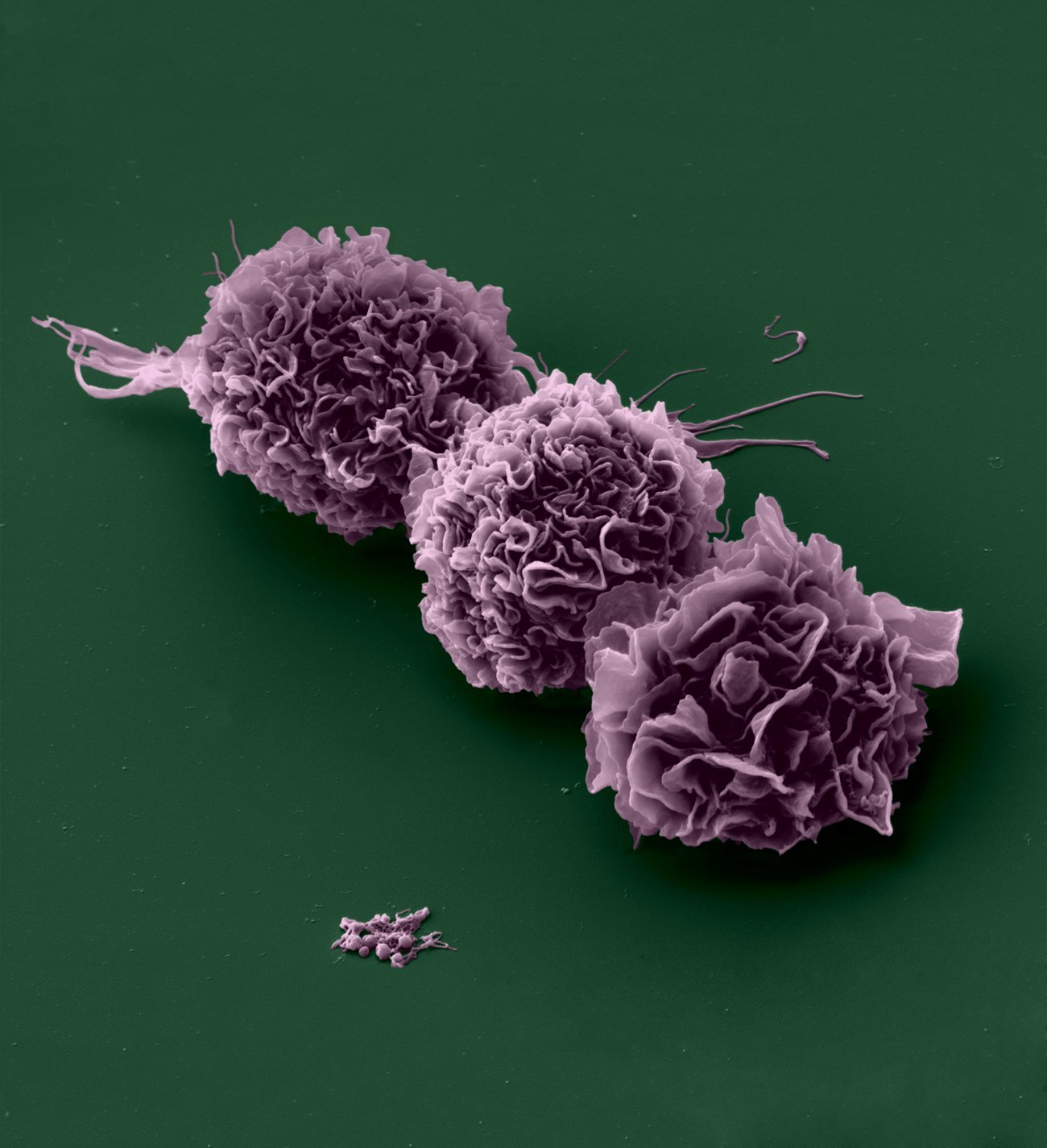
Dendritic cells - Credits : Institut Pasteur/Olivier Schwartz, Plateforme de Microscope électronique. Colorisation Jean Marc Panaud
Hepatitis B: Adapting detection and treatment
With Yusuke Shimakawa, a scientist in the Epidemiology of Emerging Diseases Unit
Hepatitis is a liver inflammation generally caused by viruses, or more rarely by toxic substances. These viral infections are triggered by five pathogens identified by the letters A to E. Hepatitis B is one of the most common human diseases – it has already affected more than two billion individuals. In Sub-Saharan Africa, a highly endemic area, scientists from the Institut Pasteur are developing screening models to optimize treatment for infected individuals and limit disease spread.
Origins
The first outbreak to be recorded probably occurred in 1883, in a shipyard in Bremen where the workers had been vaccinated against smallpox with lymph from other people carrying the as yet unidentified hepatitis B virus. The antigen that causes hepatitis B was identified in 1964 by Baruch Blumberg, a US scientist and laureate of the 1976 Nobel Prize in Medicine for his discovery.
Symptoms, transmission and treatment
The virus is transmitted through bodily fluids and secretions. The main transmission routes are from mother to child during childbirth and through sexual contact or close contact with an infected person, use of contaminated syringes and unscreened transfusions. The virus is extremely infectious and affects more than 5% of the general population in Sub-Saharan Africa, East Asia and the Pacific. Some 254 million people worldwide live with chronic HBV infection. The disease is often asymptomatic, or it may cause symptoms similar to flu or other liver inflammation. There are no specific treatments for acute hepatitis; the main defense is the body's own immune system. Chronic infection is treated with specific antivirals in people at high risk of chronic liver disease. Vaccines were developed in the early 1980s and have a very high effectiveness rate for 98% of the population.
Adaptation to the Sub-Saharan context
The most affected countries are also those with the most limited resources for screening and treatment. While many people are asymptomatic carriers of the virus, some end up developing chronic diseases that can lead to cancer. A universal approach to diagnosis and treatment may seem a good idea if access to tests determining treatment eligibility is limited. But given the cost and the difficulty in offering lifelong access to treatment, it may not be an appropriate option. The Institut Pasteur's teams have developed the first mathematical model to determine optimal eligibility for antiviral treatments for the Gambia. The result suggests a simplified, targeted strategy using the diagnostic test TREAT-B, which combines the hepatitis B e antigen (HBeAg) and alanine aminotransferase. This strategy would reduce cases of cirrhosis and hepatocellular carcinoma and would cost just half as much as a universal treatment approach.