Unraveling the brain's mysteries
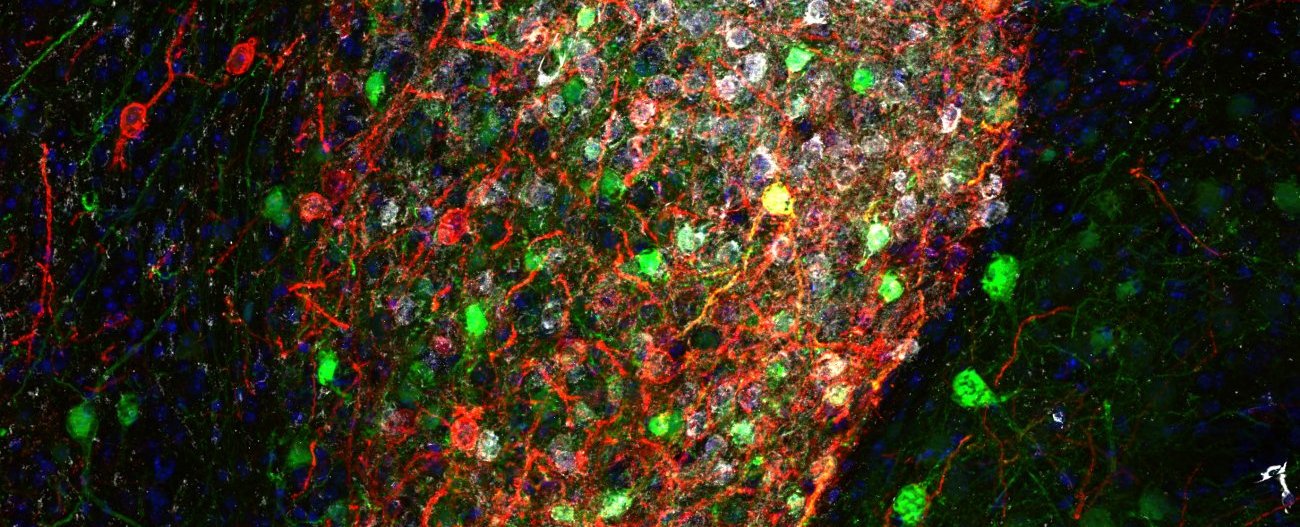
Copyright: Institut Pasteur/Ferdinand Jagot
The brain is the body's conductor, orchestrating a host of vital functions. It is in charge of our language and movements. It is the seat of our consciousness, intelligence and memory and our emotions, thoughts and perceptions. But although our understanding of the brain is improving all the time, in many ways it remains a mystery to us. At the Institut Pasteur, teams are working to understand normal brain function and also to shed light on brain dysfunction and certain brain disorders. Brain connectivity and neurodegenerative diseases are one of the priority scientific areas of the Institut Pasteur's 2019-2023 Strategic Plan.
There are 80 billion nerve cells in the brain – almost as many planets in the Milky Way* (source : The remarkable, yet not extraordinary, human brain as a scaled-up primate brain and its associated cost, PNAS). Some of these cells are neurons, but the brain also contains glial cells, which surround neurons and help control the chemical and electrical environment by providing them with nutrients and removing their waste. Neurons exchange information through synapses (see diagram), which are located at their tip and link them to other neurons.
*Correction as of September 9, 2024: we had previously written "almost as many as there are stars in the universe" instead of "almost as many planets in the Milky Way"... (at least 100 billion planets in our galaxy). Thanks to our loyal readers for pointing out the error.
The brain, a highly connected organ
How are brain circuits established before we are born? How do they change over our lifetime? We need to understand these mechanisms to shed light on brain disorders. Almost all brain disorders and conditions involve a change in the way in which neurons communicate with other cells and the brain communicates with the rest of the body. Several research centers worldwide, including the Institut Pasteur, are focusing on this aspect: "Just as scientists joined forces to elucidate the human genome, they are now coming together for an even more ambitious and complex undertaking, namely mapping the connections in the brain and understanding how the code behind the electrical activity in the brain is used to perceive and process information and generate movements, language and thoughts," explains David DiGregorio, Head of the Institut Pasteur's Synapse and Circuit Dynamics laboratory.
The brain: a complex organ interacting with its environment
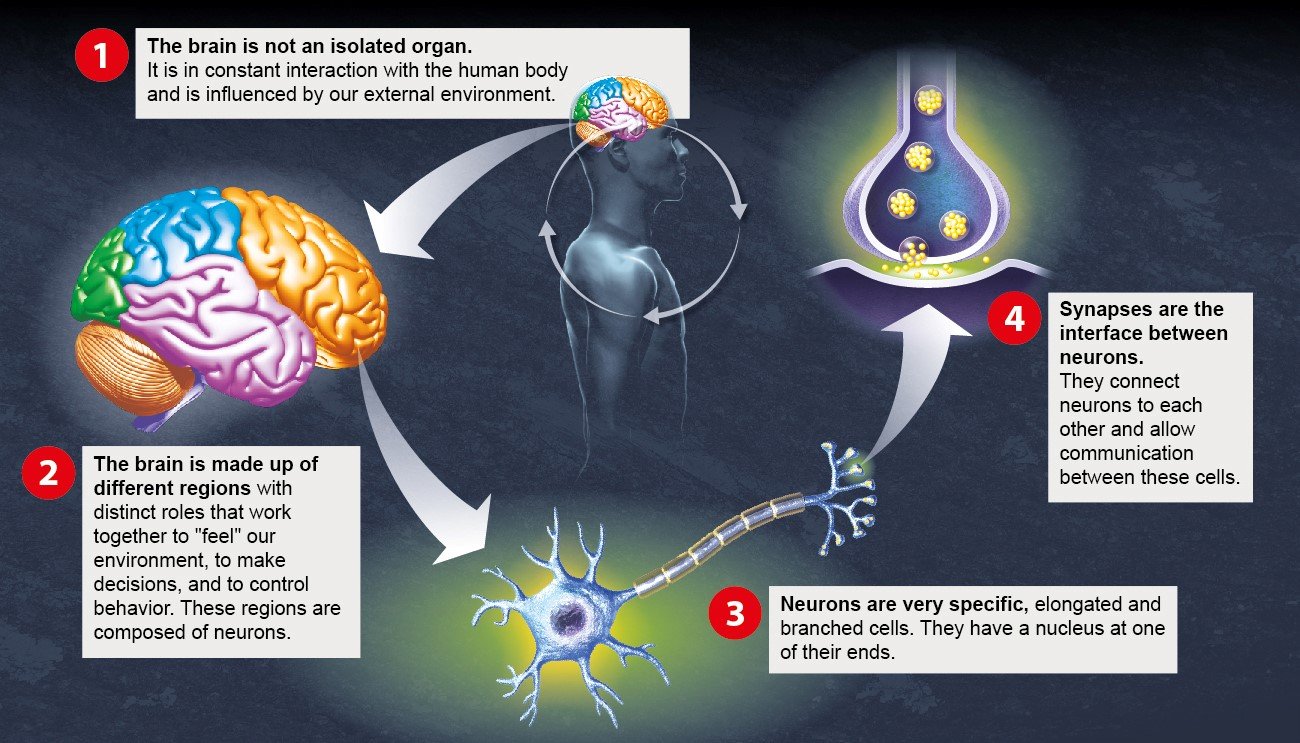
1 - The brain in its environment
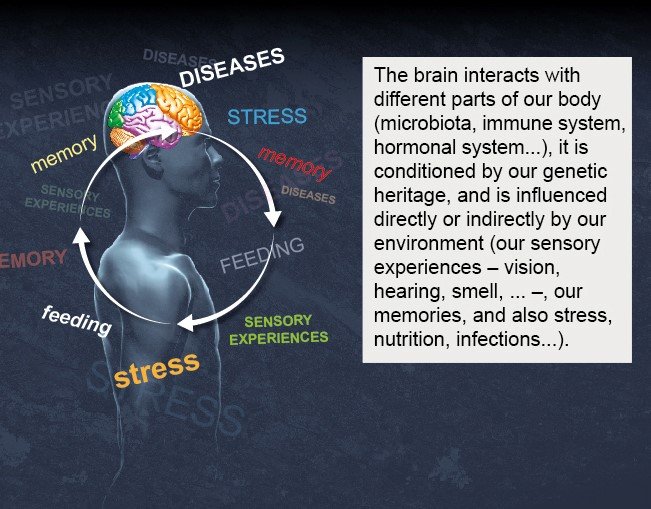
2 – Main areas of the brain
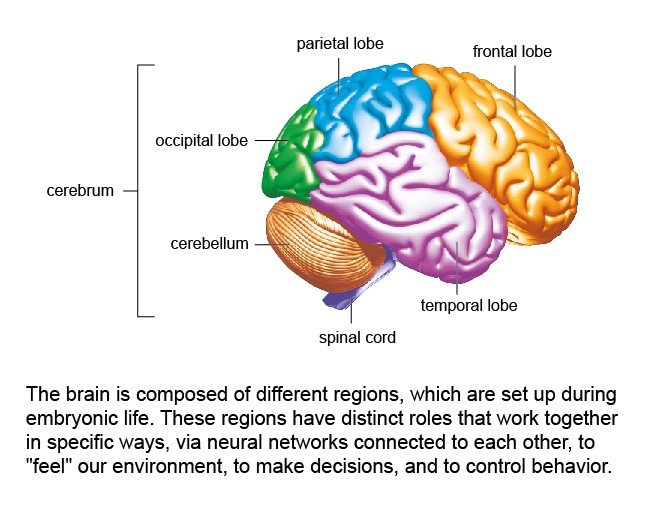
3- Neurons and their role
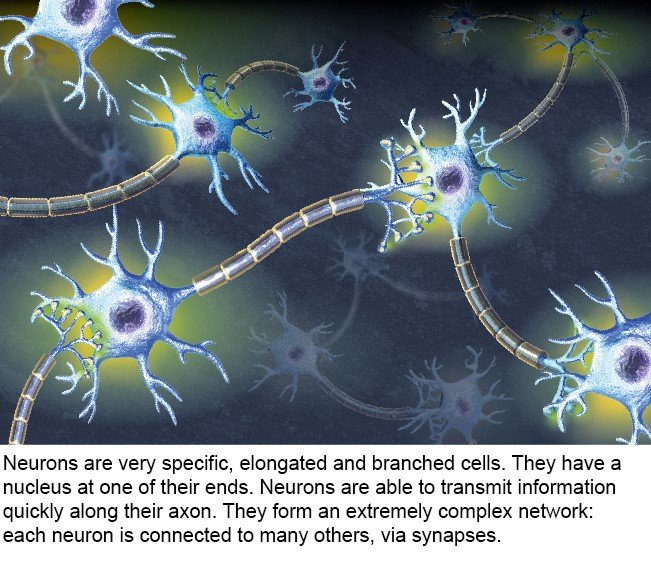
4- Synapses and their role
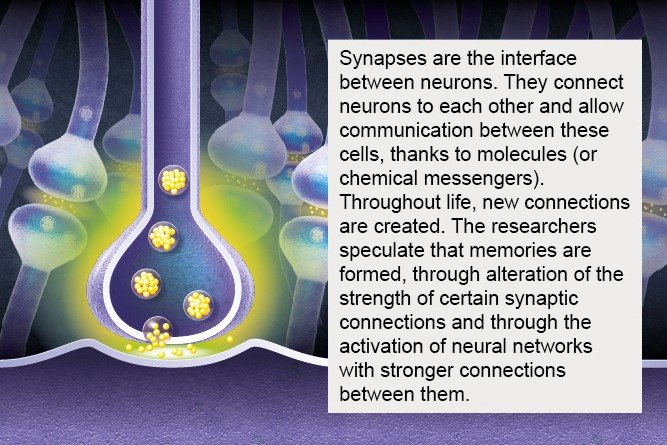
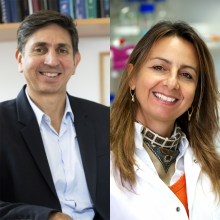
endif; ?>We will only be able to understand brain disorders and develop innovative therapies by adopting a multidisciplinary approach.
Do we know how the brain is structured?
David Digregorio :
The brain is composed of several subregions. These have traditionally been associated with specific behaviors: processing visual information, controlling movements, feeling hunger, moving through space and even the influence of emotions like fear. But it is becoming increasingly clear that the brain activity that underpins behavior involves multiple regions, comprising networks of nerve cells connected by synapses.
Unlike electrical circuits, connections between neurons are plastic, and the strength of their connectivity – in other words their ability to transmit information – can change. The brain has developed its extraordinary cognitive abilities within a body that detects the world around it. The brain safeguards the survival of the body by communicating with other systems, such as the immune system, the digestive system, the hormonal or endocrine system and the cardiovascular system. So it is thought that impaired connectivity in the brain, or between the brain and the body, is the cause of neurological, neurodegenerative and psychiatric disorders.
A particular characteristic of the human brain, as opposed to the brains of other mammal species, is that the neocortex region of the brain is relatively large [see diagram]. The neocortex is associated with multiple cognitive functions, especially producing and understanding language.
You say that the brain communicates with other parts of the body. How does it do this?
David Digregorio : The brain is not an isolated organ; it is "connected," either by direct neural connections via electrical signals or by chemical signals such as hormones, with other parts of the body that can influence essential bodily functions such as hunger/satiation and even mood. Brain function can be influenced by the state of the microbiome within the digestive system and by the immune and inflammatory systems. As we saw recently during the pandemic, even microbial infections can directly influence brain function and behavior. The Institut Pasteur has capitalized on its multidisciplinary research to establish a more holistic approach to investigating how the brain-body connection influences brain function and dysfunction.
What other brain research is going on at the Institut Pasteur?
Chiara Zurzolo :
In 2019, the Institut Pasteur management team identified "conditions associated with brain connectivity and neurodegenerative diseases" as a priority research area. The aim is to draw on the Institut Pasteur's expertise in fundamental research in neuroscience, genetics, cell biology and development, immunology, microbiology and biology of infection to improve our understanding of the complexity of brain function and brain disorders. This program is led by David DiGregorio, a neuroscientist, and myself, a cell biologist, and we have a steering committee whose members include developmental biologists, immunologists and cell biologists, as well as neuroscientists with different specializations.
This support for our research, as provided for in the Institut Pasteur's Strategic Plan, is a unique opportunity for the Institut Pasteur to apply its scientific expertise to some of the major questions in neuroscience: understanding how the brain works, elucidating what doesn't work in diseases such as Alzheimer's, Parkinson's, autism and schizophrenia, and developing innovative treatments. The failure of several costly clinical trials highlights the need for a deeper understanding of the mechanisms behind these conditions and a fresh approach. We will only be able to understand brain disorders and develop innovative therapies by adopting a multidisciplinary approach.
How does the brain work?
Scientists can only treat brain disorders if they understand how the brain works. The origins of disease vary hugely and it is therefore essential when studying any organ – including the brain – to consider every level, from the molecule to the whole body and the surrounding environment.
The Institut Pasteur's Synapse and Circuit Dynamics laboratory has embarked on an ambitious challenge: to understand how behavior is formed by the biological processes generated by molecules, synapses, neurons and chains of connected neurons known as neural circuits. As David DiGregorio, who heads the laboratory, explains, "We want to understand how sub-second time [less than a second] is encoded in the brain to generate skillful movements that involve interaction with the external environment (such as catching prey or playing the piano). We put forward the theory that the brain has developed internal clocks." In 2019, the team's scientists showed how the nanoscale organization of synaptic molecules drives diversity in communication between neurons. This characteristic of synapses also seems to enable the representation of time, which is used to generate precise movements.
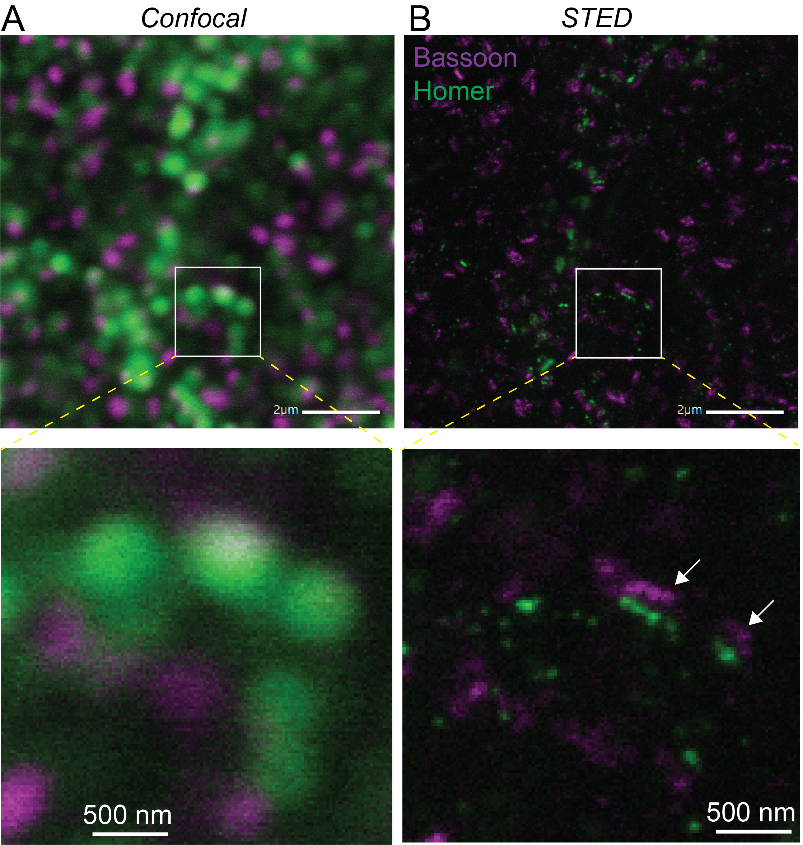
Microscopes are an essential tool for biologists. They can be used to observe cells – the basic living component of biological organisms – and also several fundamental processes involved in life that are invisible to the naked eye.
Cells vary in size from 1 to 50 millionths of a millimeter. But their constituent molecules which are responsible for multiple chemical reactions essential for life are closer in size to a nanometer or less. Two types of microscope are therefore needed to observe living phenomena: optical microscopy is used to observe processes as small as just under half a micrometer, or 500 nanometers. But electron microscopes are needed to see cellular structures at single-molecule resolution, or in some cases even at atomic resolution.
In addition to these two conventional microscopes, two new techniques are improving our ability to visualize living systems. Super-resolution fluorescence microscopy (recognized with a Nobel Prize in 2014) is used by neuroscientists to observe the brain's processes and molecular organization at resolutions down to 10 nm. In 2017, a Nobel Prize was also awarded for cryo-electron microscopy, an extraordinary technique used to visualize single proteins within cells and even the structure of the proteins themselves. Scientists at the Institut Pasteur have access to these two technologies, which offer an unprecedented level of detail when viewing synapses and brain cells. Other technologies exist such as the Titan KriosTM microscope, an electron microscope equipped with extraordinary capabilities (read below the use of Titan KriosTM in the study of long Covid).
But despite these advances, our ability to observe brain cells in their natural environment is hindered by the opacity of brain tissue. To overcome this obstacle, neuroscientists use a technique known as multiphoton excitation fluorescence microscopy. This microscopy method has revolutionized our understanding of how individual neurons and neural networks function in experimental samples and, in some cases, in animals. The technology is in use in several laboratories and shared facilities at the Institut Pasteur.
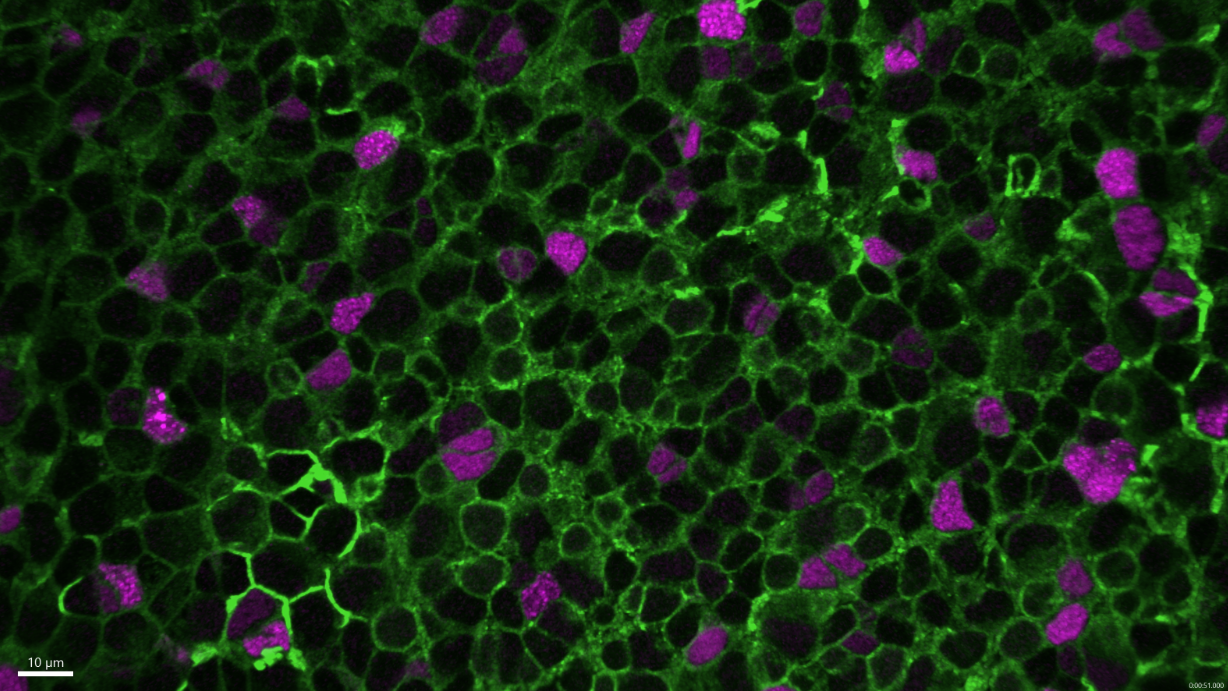
Neural stem cells (Zebrafish brain). Confocal microscopy.
Copyright: Institut Pasteur/Nicolas Dray, Laure Mancini, Laure Bally-Cuif
How does the brain develop?
The first stage in brain formation occurs in the fetus, around day 19 of pregnancy, when cells form a neural plate and then a neural tube. The plate is formed from a group of embryonic cells known as the neuroectoderm. At this stage, the brain is still under development; it is not yet mature enough to give instructions or receive information.
"There are stages in cell regulation, interaction and migration which cause the embryo, initially a ball of cells, to identify a predefined region to form the brain," explains Laure Bally-Cuif, Head of the Institut Pasteur's Zebrafish Neurogenetics laboratory. The cells in this area then multiply and the region grows and then regionalizes: the structure acquires an orientation, in other words a front and rear. Additional subdivisions within the region then help create brain areas, and each area acquires a different identity. The border zones between each area are crucial for the precise regionalization of the brain, since they emit signals with organizing properties. "At this stage of development, the organization of the brain is the same in all vertebrates. It is hard to tell the difference between a mouse, fish, tortoise or chicken brain!" smiles Laure Bally-Cuif.
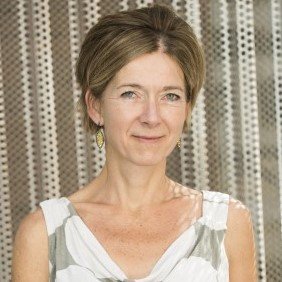
At this stage of development, the organization of the brain is the same in all vertebrates. It is hard to tell the difference between a mouse, fish, tortoise or chicken brain!
Laure Bally-CuifHead of the Institut Pasteur's Zebrafish Neurogenetics laboratory
Once these areas have been formed, neurogenesis begins. This involves the progressive differentiation of cells, which are still progenitor cells, in these areas. Progenitor cells become either neurons or glial cells, the other cell type in the brain. When they differentiate, the cells acquire functional properties, enabling them to execute their different roles. But some cells remain as progenitor cells and persist over the long term. These cells can give rise to new neurons even in adulthood. They are known as stem cells. The way in which these cells are selected and maintain their properties until adulthood remains a mystery.
During neurogenesis, different neurons are produced in each brain area. It is at this stage that the brains of vertebrates start to differ from each other, especially in terms of morphology and size. In humans, for example, the cortex is much larger than the other brain areas. In fish, the cerebellum grows the most during development.
Understanding the border zones in the brain and their roles in disease
All of the processes involved in early brain development are highly sensitive to multiple factors (genetics, toxins, infections, etc.). Abnormalities can give rise to neurodevelopmental syndromes, some of which can be very severe and affect brain size or function, for example the ability to control behavior.
Brain development also takes place in conjunction with other tissues and organs that are being formed.
"Our research investigates the choroid plexus, the interface between the blood and nervous system, which enables dialog between these tissues. It can receive external signals and produce factors that influence the brain," explains Aleksandra Deczkowska, a scientist in the Institut Pasteur's Brain-Immune Communication group.
It is also involved in aging – as the body ages, the choroid plexus starts producing antiviral signals, and this has a negative impact on the brain.
Autism – a heterogeneous disorder with a genetic component
Autism affects more than 650,000 people in France. It is a highly heterogeneous syndrome but is characterized by two main criteria: atypical social interaction and restricted and/or stereotyped behaviors and interests. Apart from these criteria, however, the symptoms and impact on the lives of those concerned can vary considerably and may include intellectual disability; sensory hypersensitivity or hyposensitivity; emotional distress at changes in environment; sleep disorders; epilepsy; and hyperactivity. The heterogeneous nature of autism makes research complex and the causes of the disorder hard to pinpoint.
But scientists are now in agreement that it has a strong genetic basis. It was at the Institut Pasteur that the first mutations involved in autism were identified in 2003, by the Human Genetics and Cognitive Functions Unit led by Thomas Bourgeron, in collaboration with psychiatrists Marion Leboyer from France and Christopher Gillberg from Sweden. Since then, the unit has continued to study the genetic component of autism. The work of these scientists and several other teams worldwide has led to the identification of several genetic mutations. But these mutations are not always hereditary; in 10-20% of cases they are de novo mutations which occur after birth.
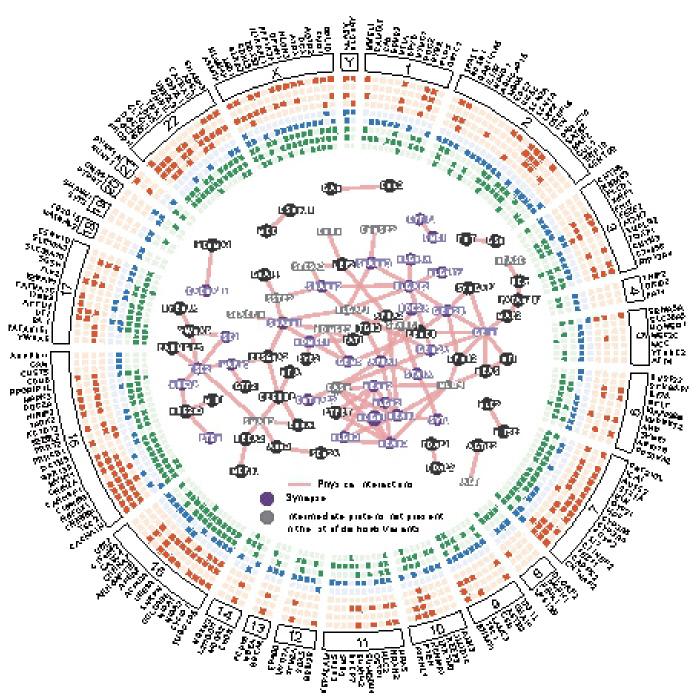
The Applied and Theoretical Neuroanatomy group, led by Roberto Toro at the Institut Pasteur, explores the development and evolution of brain anatomy. The team uses mathematical modeling methods, magnetic resonance imaging and genomics. "We develop a wide variety of methods, which we use to understand the natural diversity of the human brain and to identify differences associated with neurodevelopmental disorders," explains Roberto Toro. The group particularly focuses on autism.
Reconstruction of human brain connectivity obtained from diffusion-weighted magnetic resonance imaging data. Copyright: Institut Pasteur/Roberto Toro
Simulation of brain anatomy development with a biomechanical model. Top: simulation from an ellipsoid. Bottom: simulation of the development of a Ferret brain. Copyright: Roberto Toro.
3D reconstruction of the anatomy of the cerebral cortex of 34 primate species for the study of brain evolution. Copyright: Katja Heuer et Roberto Toro (DOI: 10.1016/j.cortex.2019.04.011).
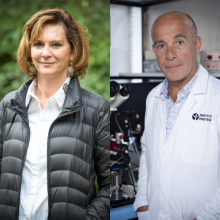
endif; ?>Our research shows that it is very important to test emotional biases in animals, especially if we want to test drugs and molecules that are intended to reverse these biases.
Which animal model is currently used to study depression?
Chantal Henry: We use what are known as "depression-like" mice, which have the same symptoms and biological parameters as humans with depression. They are less active, they make less effort to search for food and take less good care of themselves. For example, if we rub them with a sugar water solution they won't clean themselves as they normally would.
Pierre-Marie Lledo: There is also a loss of pleasure – if the mice have access to both fresh water and a sugar water solution, they won't show a preference for the sugar solution. By reducing depression to biological abnormalities (blood factors and dysfunction in neural circuits that are found in both rodents and humans), we can try to elucidate the roots of depression by studying rodents.
How do the mice acquire these symptoms?
Pierre-Marie Lledo: The mice become "depression-like" when they are placed in an unpredictable environment with multiple changes that they have no control over and can only observe; for example, if their living conditions change every day and they have to deal with various threats (a shortage of water or food, social isolation, changes to the light cycle, etc.). Like humans, when animals are faced with chronic adversity they produce hormones that help the body to adapt, like high levels of cortisol, or glucocorticoids in mice.
Why is it difficult to study depression in mice, and how can these difficulties be overcome?
Chantal Henry: That is what we wanted to investigate. In mood disorders, whether depressive episodes or manic excitement, there is a perception bias. In depression, pleasant stimuli become unpleasant, and unpleasant stimuli become even more so – there is a negative emotional bias. In mice with a depression-like phenotype,* the motor aspect has often been investigated, but the emotional bias is studied more rarely.
*Phénotype: The set of observable characteristics of an individual, corresponding to the expression of the genotype.
Pierre-Marie Lledo: Research in behavioral science using animal models has long been hampered by the simplistic view that mice have no mental state, intentions or affect (the ability to feel emotions or moods). We wanted to incorporate the impact of affect into our research.
Chantal Henry: To investigate the presence of affect in animal models, we made the mice smell positive, neutral or negative scents without any prior learning experience. Compared with the other mice, those with a depression-like phenotype spent less time on the positive, pleasant scents, and even less on the negative scent. This confirmed the existence of a negative perception bias.
Why do these mice make a good model for studying depression?
Chantal Henry: Taking emotional biases into account, we demonstrated that there had been a change in the circuitry involving certain neurons in a subregion of the amygdala (the part of the brain containing the system for managing and generating emotions). We observed a change in activity in the neurons that generally code for pleasant or unpleasant stimuli; the neurons that code for unpleasant stimuli were more active.
This confirms that mice are a useful animal model for studying depression. In the mouse model with a manic-like phenotype, however, we found no negative emotional bias, which leads us to believe that it is not a suitable model for research into this disorder.
Our research shows that it is very important to test emotional biases in animals, especially if we want to test drugs and molecules that are intended to reverse these biases.
How does the brain age?
As we get older, our brain also ages. Tasks like memorizing things or learning new skills can become more difficult with age. This is caused by a variety of factors such as the shrinkage of some brain areas and a reduction in the number of connections between neurons. Diseases like Alzheimer's and Parkinson's also lead to the degeneration of some neurons because of an accumulation of misfolded proteins. By living healthily and remaining physically, socially and intellectually active we can reduce the risks associated with aging, but we cannot eliminate them entirely. One of the Institut Pasteur's missions is to further our understanding of the aging process and age-related disorders with the aim of improving treatment.
Understanding memory
Improving our memory involves understanding how it works. But many aspects of memory function remain a mystery. Institut Pasteur scientists set out to investigate how new memories are formed.
"If you visit the Eiffel Tower, for example, you form an initial memory. Then if you visit it again, several things have to happen: you need to remember your first visit, and at the same time form a separate new memory. It was never clear how the brain managed to do this," explains Christoph Schmidt-Hieber, Head of the Institut Pasteur's Neural Circuits for Spatial Navigation and Memory group.
In 2020, the scientists used mice to observe the involvement of the gateway to the hippocampus, the part of the brain that is particularly important for the formation of episodic memories – in other words memories of specific events (read Un air de déjà-vu ? Comment se forment les souvenirs distincts...). This gateway region detected even small changes to the environment, whether it was unknown or familiar. The study demonstrated the role of the hippocampus in the formation of distinct memories. More recently, a group of scientists including Christoph Schmidt-Hieber identified the specific signal emitted in the hippocampus that is responsible for identifying a memory as a new one.
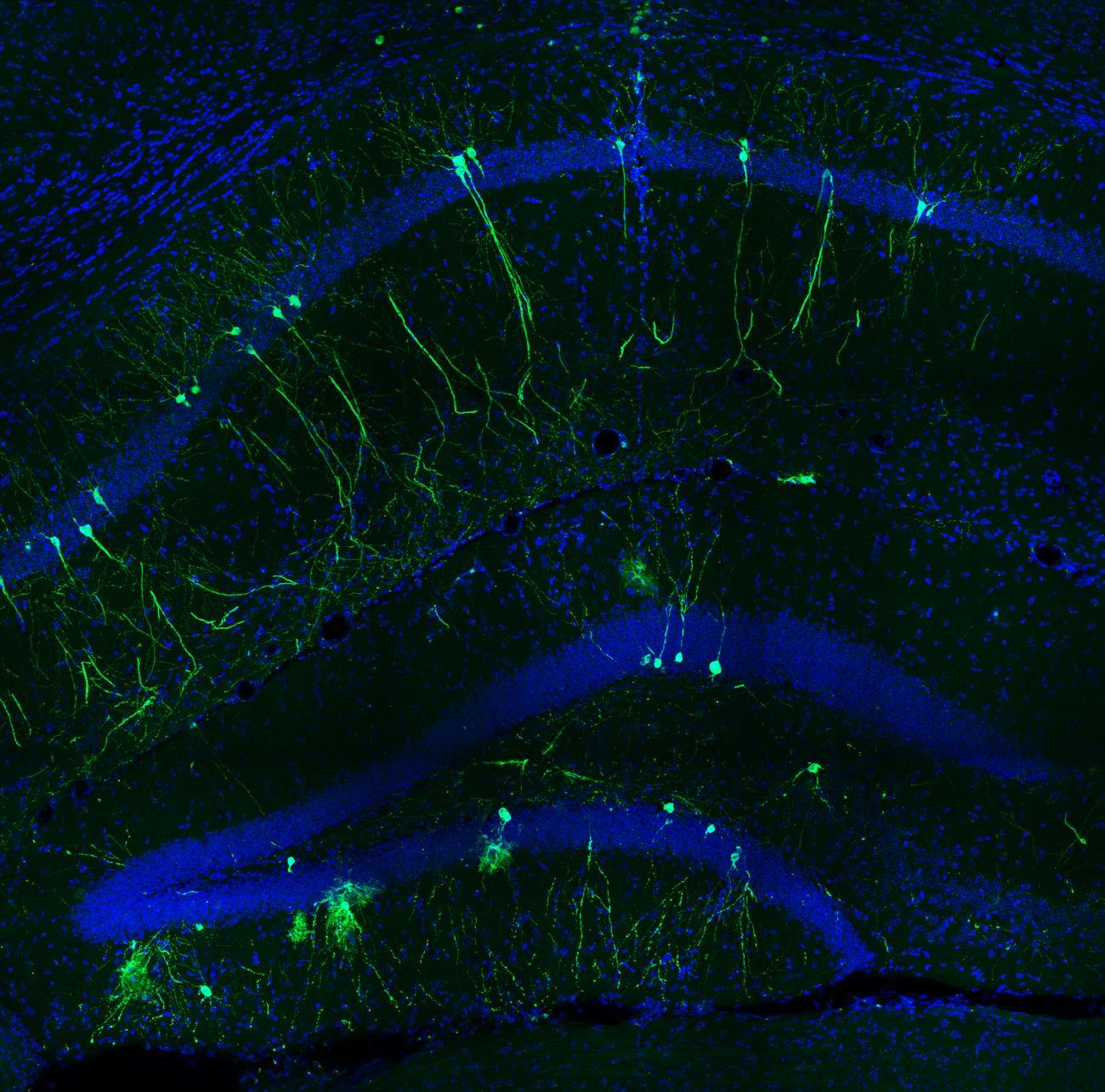
Elucidating age-related diseases
Alzheimer's and Parkinson's are among the best known age-related diseases. These two neurodegenerative diseases have many points in common. They are both characterized by neuron degeneration: dopaminergic neurons in the substantia nigra in the brain for Parkinson's, and neurons in various regions including the hippocampus – the area responsible for long-term memory – for Alzheimer's. Another common feature of the two conditions is the role of misfolded proteins in the spread of the disease: tau proteins for Alzheimer's and α-Synuclein proteins for Parkinson's. These misfolded proteins spread through the brain and form aggregates.
"Elucidating how the proteins spread and form aggregates is crucial for our understanding and treatment of these diseases," explains Chiara Zurzolo. The scientist leads the Institut Pasteur's Membrane Traffic and Pathogenesis Unit, which has recently revealed two mechanisms. First, the scientists elucidated the way in which tau and α-Synuclein proteins spread between neurons. The team also showed that lysosomes are involved in the spread of α-Synuclein proteins in Parkinson's disease and that tau proteins are able to evade degradation in Alzheimer's disease. These discoveries may pave the way for new therapeutic targets.
In Alzheimer's disease, as well as tau protein aggregates there are also aggregates composed of other proteins known as amyloid beta proteins. The Integrative Neurobiology of Cholinergic Systems Unit has developed a model that expresses human amyloid beta proteins to facilitate research into Alzheimer's disease. They also use human neurons derived from induced pluripotent stem cells (iPSCs) and transplant them into an in vivo model.
Exploring the interaction between immunity and the brain
There is considerable evidence that the immune system interacts with the brain. When we are ill, the brain reacts to infection and we "feel" ill. Conversely, brain lesions can cause abnormal immune responses. "In mice, we have noticed that an absence of T cells, a type of white blood cell, leads to impaired cognitive abilities. Similarly, mice lacking interferon-gamma, a crucial antiviral factor, become antisocial," explains Aleksandra Deczkowska, Head of the Institut Pasteur's Brain-Immune Communication laboratory.
But little is known about the mechanisms behind these interactions. Aleksandra Deczkowska particularly focuses on microglial cells and the role of immunity in brain aging. Microglial cells seem to be particularly involved in Alzheimer's disease – the microglial cells around amyloid plaques express highly specific genes, which suggests that these cells can serve as a defense system against the disease. "Our laboratory is now trying to shed light on the factors involved in the formation of these cells and whether they can be strengthened to prevent Alzheimer's disease," concludes Aleksandra Deczkowska.
The search for an elixir of youth
This elusive quest is the focus of Lida Katsimpardi's research. When she was at Harvard, the scientist discovered that transferring the blood of young mice to older mice reduced the effects of aging by influencing neuron production and stimulating vascular remodeling. She identified a compound involved in this effect, known as GDF11. Lida is now an Inserm scientist in the Institut Pasteur's Perception and Memory Unit and the Institut Necker-Enfants Malades, and together with her colleagues she recently elucidated the role of this protein, which is similar to the effect of calorie restriction.
After being infected with the SARS-CoV-2 virus, some COVID patients continue to suffer from symptoms of varying severity for several months. This is known as long COVID. The Institut Pasteur is developing tools to shed light on the reasons for COVID persistence. Scientists in the Institut Pasteur's Membrane Traffic and Pathogenesis Unit, led by Chiara Zurzolo, have discovered that SARS-Cov-2 is not capable of infecting neurons directly; it can only access them via virus-permissive cells like epithelial cells. This discovery combines both the study of in vitro cultures, showing that healthy neurons are infected if they come into contact with infected cells, and the use of state-of-the-art microscopy tools, such as the Titan KriosTM microscope from the Institut Pasteur's Nanoimaging platform, which makes it possible to obtain unprecedented resolution of biological samples and nanomolecules, closer to actual biological conditions.
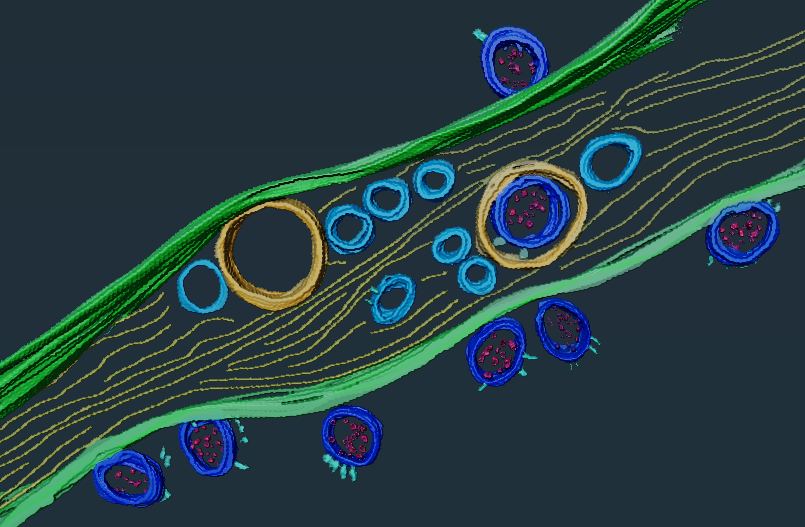
This discovery also supports the theories put forward by other scientists at the Institut Pasteur that the virus reaches the brain via cells in the olfactory epithelium. To elucidate this mechanism, the Institut Pasteur's scientists are developing a system to use two-photon excitation microscopy. This technique enables scientists to obtain images of tissue up to a millimeter deep. "With this microscope, we can study the brain activity of mice when they are infected with SARS-CoV-2 or other viruses. We want to see how an active infection changes the activity of both neurons in the brain and also non-neuronal cells such as microglial cells. We can then observe whether this brain activity is still activated after infection," explains Uwe Maskos, Head of the Integrative Neurobiology of Cholinergic Systems laboratory. The microscope will be installed in a BSL3 laboratory for this research. To understand how SARS-CoV-2 penetrates the brain, and how long it remains there, the scientists are planning to use a fluorescent version of the virus. They have already demonstrated that human neurons in culture are liable to be infected with the virus if the ACE2 protein, the gateway to the cell, is sufficiently expressed.
New therapeutic targets
Understanding how the brain works and how it interacts with different parts of the body helps improve not just the identification but also the treatment of diseases. Each new mechanism discovered can pave the way for a future drug.
From nicotinic receptors to new drug discovery
Nicotinic receptors are molecules that have many crucial roles to play in the nervous system: they are involved in neuromuscular transmission, pain, attention, memory, etc. But they are also implicated in addiction, especially nicotine and cocaine addiction. Developing a better understanding of the structures and mechanisms of nicotinic receptors may therefore lead to the identification of new drugs to treat these addictions.
"Nicotinic receptors are composed of five subunits. Some have five different subunits and include the α5 subunit, while others have five of the same subunit, known as α7," explains Pierre-Jean Corringer, a scientist in the Genes, Synapses and Cognition joint research unit at the Institut Pasteur. These two receptor subtypes are the focus of his team's research. "Scientists have only recently begun to study α5, since it is not essential to receptor function. But in the 2000s, genome analysis showed that a specific mutation in α5 caused much higher than average susceptibility to nicotine addiction and lung cancer."
In 2018, Institut Pasteur scientists, in collaboration with the CNRS, Sorbonne University and Inserm, showed in rats that this mutation is also involved in relapse after smoking cessation. Furthermore, the mutation also influences cocaine addiction.
Interview with Uwe Maskos : Why is it so hard to quit smoking?
Drug molecules targeting the α5 subunit could therefore have an impact on these addictions. The challenge for the Genes, Synapses and Cognition joint research unit is now to find molecules that specifically target α5 without affecting the rest of the nicotinic receptor. "These molecules could have multiple applications, since the α5 mutation is behind a whole host of problems – not only nicotine and cocaine addiction but also alcohol addiction and a general effect on the reward system," concludes Pierre-Jean Corringer.
Finding compounds that target α7 could also have a significant impact, since this subunit plays a major role in cognition.
Microbiota and depression: hope for new treatments?
As we still know little about the biological mechanisms behind depression, it can be difficult to devise a suitable treatment plan. But the discovery that the microbiota is involved could lead to new treatments.
Scientists at the Institut Pasteur have discovered that when the microbiota of a mouse used as a model for depression is transferred to another mouse, the latter also shows signs of depression. "We have observed that depression-like behaviors can modify the gut microbiota and its functions. It is not yet clear how these behaviors affect the microbiota, but we know that the immune system, which controls the microbiota, is negatively affected by depression," says Gérard Eberl, Head of the Microenvironment and Immunity Unit. Some bacteria, including lactobacilli, are lacking in the microbiota of mice with depression-like behaviors.
The scientists then observed that a change in the gut microbiota leads to a lack of some compounds in the blood, including tryptophan, diacylglycerol and arachidonic acid. Tryptophan is the basis of serotonin, the hormone that is missing in depression. Diacylglycerol is the basic component of mediators that play a key role in the immune system and the brain, especially endocannabinoids, which are crucial for mood regulation. If confirmed in humans, this discovery could improve treatment for depression. "Depression has a range of causes, but our discovery could identify patients who suffer from a lack of tryptophan or arachidonic acid in the blood. In these cases, we could provide them with a supplement of these compounds to reduce the risk of depression."