From Molecular Biology to Stem Cells
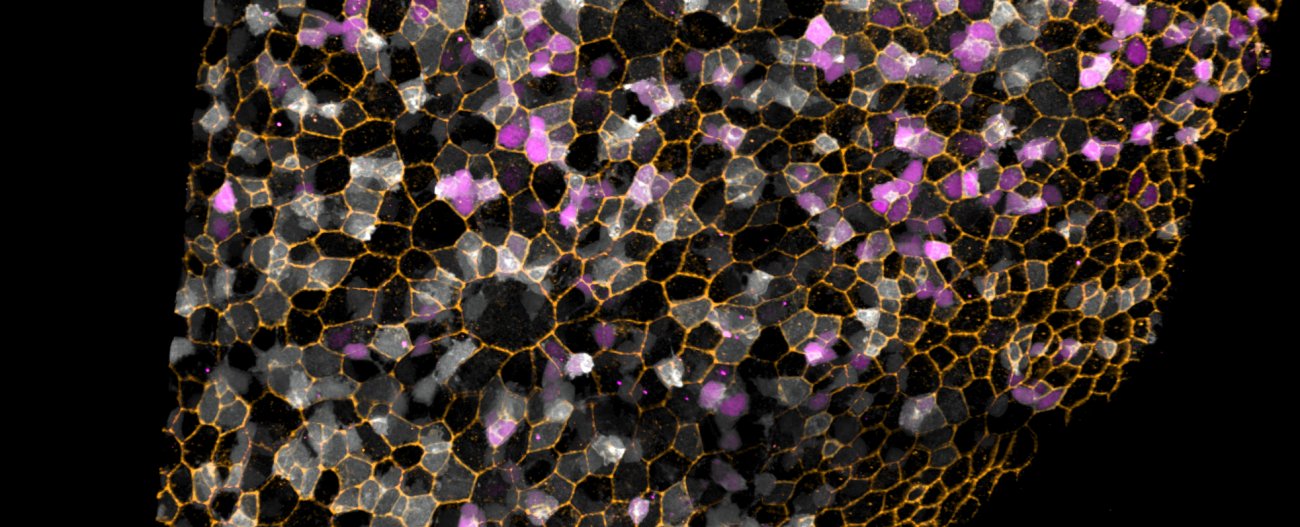
May 1968… While students took to the streets of Paris, Jacques Monod and François Jacob were getting ready for what would prove to be a decisive turning point for the Institut Pasteur: the opening of a new department dedicated to molecular biology—in other words the study of the molecular mechanisms governing cell behavior. The venture, which included plans for purpose-built premises, had recently been approved by the Institut Pasteur Board of Directors and was fully in line with the discovery that had earned Jacques Monod, François Jacob and André Lwoff the Nobel Prize in 1965. The 1950s had seen the birth of a new understanding of how genes— sections of chromosomes that each carry a specific hereditary trait—govern the synthesis of proteins, the molecules that play a critical role in the function of living beings. The three Institut Pasteur biologists demonstrated the existence of mechanisms for regulating gene expression: they showed how proteins, in turn, regulate gene activity. It was a natural progression for them to dedicate a department to the study of these regulation mechanisms, which had been discovered in bacteria but which, according to Jacques Monod, were valid in equal measure for bacteria and elephants.
Lwoff, Monod, Jacob: three Institut Pasteur scientists behind the development of molecular biology
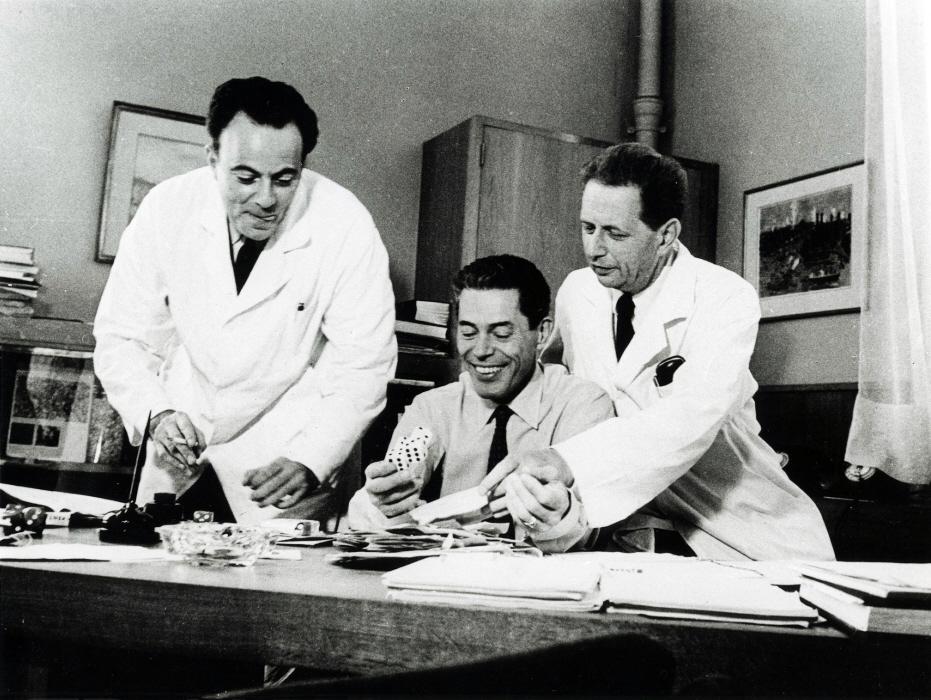
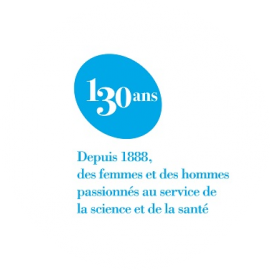
In 1965, the Nobel Prize in Physiology or Medicine was awarded to André Lwoff, Jacques Monod and François Jacob “for their discoveries concerning genetic control of enzyme and virus synthesis”. These discoveries can be summed up in one word, operon— the name given to the gene activity regulation system that the three biologists from the Institut Pasteur and their team revealed in the “attic”, the hundred or so square meters under the rafters of the Duclaux building that they used as a laboratory. In the late 1950s, scientists had recently discovered that the DNA molecule in a cell carries genetic information that is passed down from one generation to the next. It was also understood that genes play an essential role in determining the characteristics of living organisms.
But one question remained: how could cells carrying the same genes have different functions? Lwoff, Jacob and Monod solved the mystery by introducing the notion of regulatory genes. By comparing two observed phenomena—one in bacterial cultures, the other during infection of bacteria by viruses (bacteriophages)—the biologists showed that the genes of one cell are not always active. Their activity depends on other genes, called regulators, which encode proteins, which in turn inhibit, or conversely, activate groups of genes involved in the same function, sugar digestion for example. An operon is the term used by Jacob and Monod to describe a set of genes subject to the same regulation. This discovery helped to revolutionize biology by bringing it into the molecular era.
François Jacob set to work on eukaryotes
When the department opened, most of the units were researching the regulation of gene expression. Several teams continued to explore this aspect in bacteria, but François Jacob was at last able to fulfill his long-harbored aspiration: to study these mechanisms in more complex organisms—eukaryotes, which are cells with a nucleus—and, in particular, animals, including humans. Jacob was convinced that mechanisms similar to those observed in bacteria were at work in eukaryotes and that they held the key to the mystery of embryonic development—the formation of a complex organism with several organs, each with its own functions and characteristics, derived from a single cell. How was this cell differentiation possible?
But this was an era with limited tools on offer for studying embryonic development and cell differentiation. It was possible to preserve an early mouse embryo in culture for several days, at a stage where it was only a few cells large, but this systems were highly inadequate for studying what happens in tissue, for example. Nevertheless, a discovery made in the 1950s and 1960s captured Jacob’s attention: two Americans—Leroy Stevens and Barry Pierce—selected a line of mice in which a particular type of tumor, known as a teratocarcinoma, developed spontaneously in the testicles. These tumors contain many different cell types—neurons, muscle cells, skin cells, etc., but without any organization. Stevens and Pierce showed that these tumors are transplantable: even a single tumor cell can give rise to a teratocarcinoma in another mouse. The tumor therefore contains cells that are able to differentiate into several different types just like embryonic cells. The two biologists had just proved the existence—in this case in a tumor—of what would later be called pluripotent stem cells.
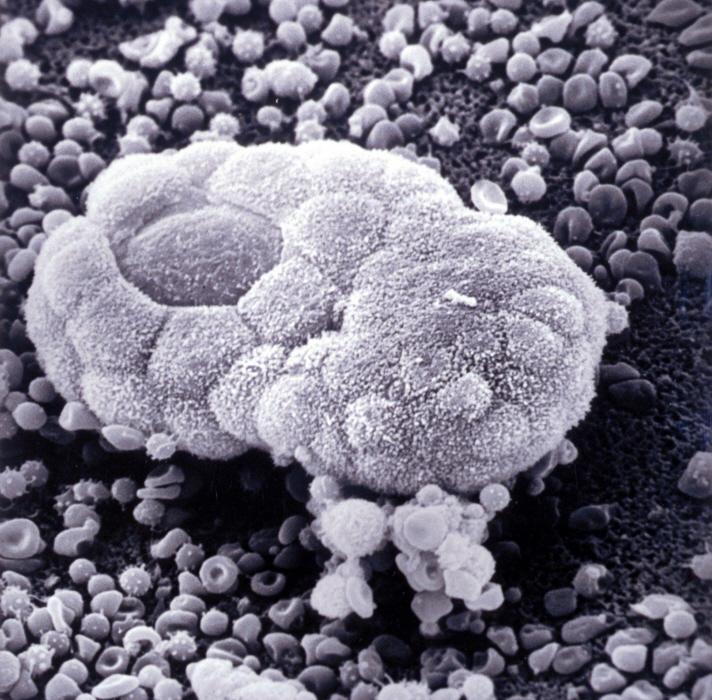
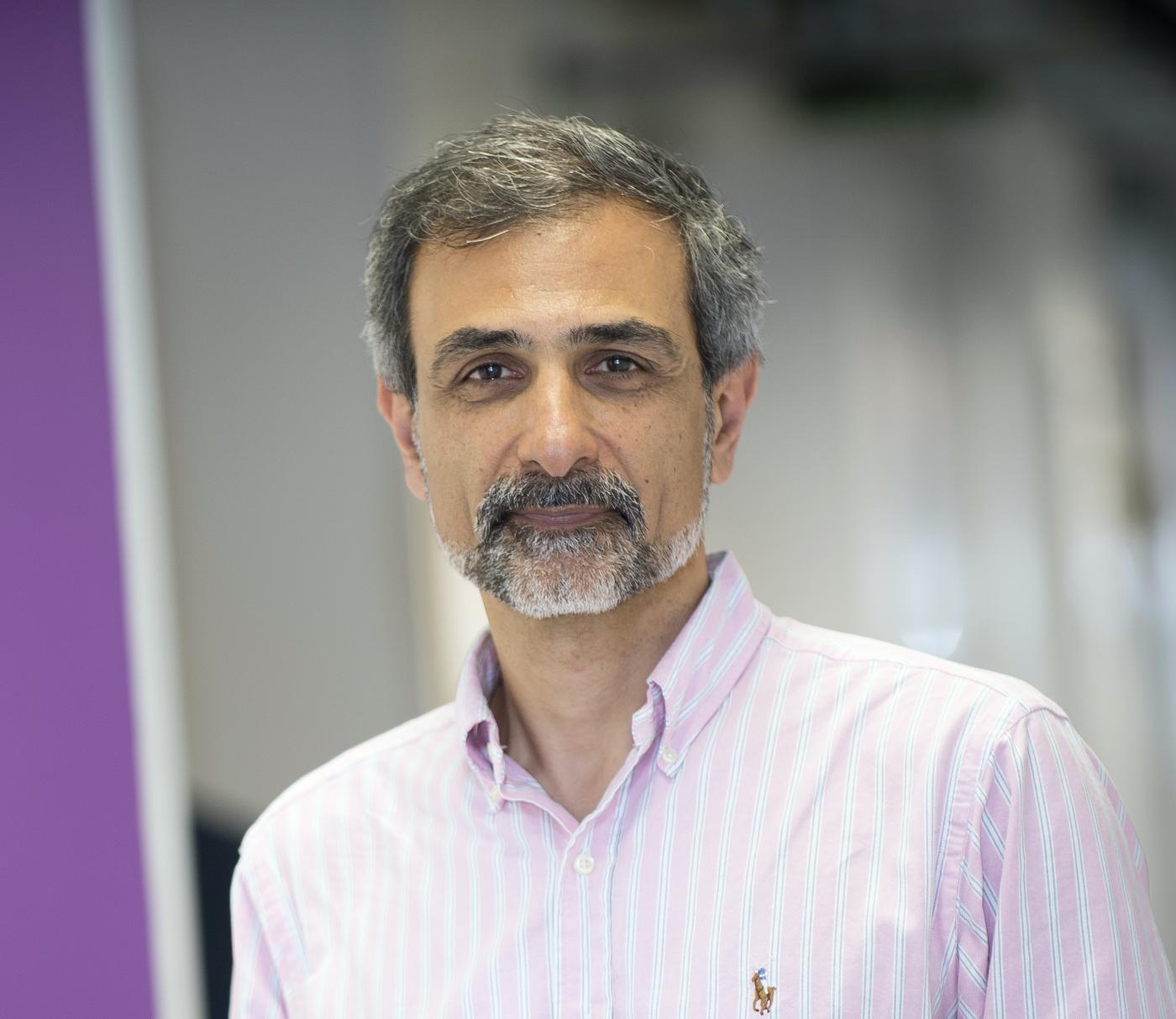
Shahragim Tajbakhsh, Head of the Stem Cells and Development Unit at the Institut Pasteur
The Department of Molecular Biology was a new departure for the Institut Pasteur because its line of research. François Jacob and Jacques Monod already had a vision of a different kind, with a focus on genes and how they were regulated in cells and tissues.
Vital contributions helped consolidate the discipline
Over time, embryologists such as Boris Ephrussi in the brand new Center for Molecular Genetics in Gif-sur-Yvette isolated these stem cells and showed their potential in the study of differentiation and the first stages of embryogenesis. Ephrussi passed on to François Jacob both his expertise and the cell lines he had produced. During the 1970s, Jacob’s team—in particular Charles Babinet, Philippe Brûlet, Jean-François Nicolas, Philip Avner and Jean-Louis Guénet—, and then others in the UK and USA, established culturable cell lines that could produce all tissue types, including heart, muscle, nerve, and skin cells.
The early days proved difficult. Even though the team had a system for studying cell differentiation in culture, there were no tools to help them understand what takes place in these cells at the molecular level. François Gros, who joined the department with Margaret Buckingham, a young Scottish recruit, to focus on skeletal muscle-forming cells, faced the same problem. However, radical changes took place from the 1970s onwards, with the introduction of new molecular biology techniques. It became possible to isolate genes coding for specific proteins, to study their position on human and mouse chromosomes and their expression during differentiation, andeven to begin studying the mechanisms for regulating their expression.
Henri Buc’s team carried out physicochemical and enzymology research and investigated protein-DNA interactions to elucidate the regulatory mechanisms of gene expression. This research was complemented by the work of Daniel Louvard’s team, which explored and promoted the discipline of cell biology within the department.
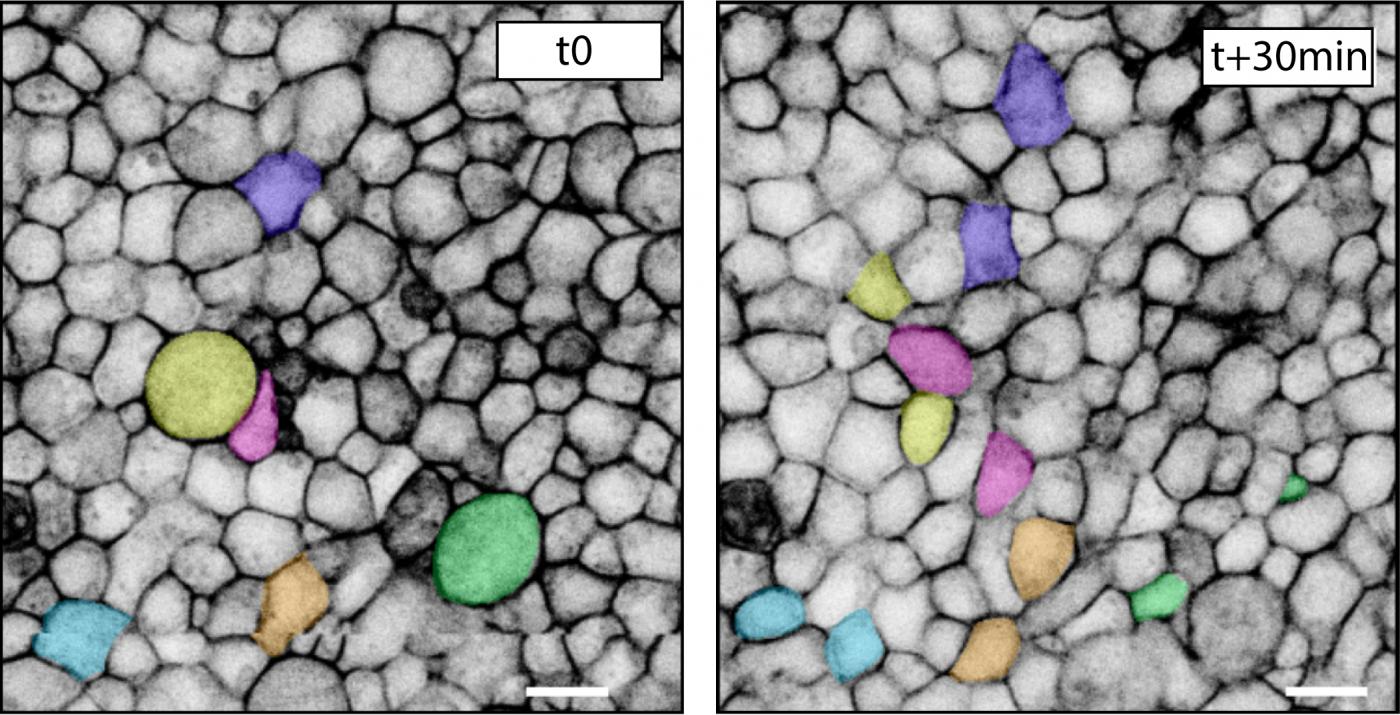
Meanwhile, research on gene regulation continued, with Anthony Pugsley and Bernard Dujon investigating single-cell models of bacteria and yeast, respectively. Their efforts supplemented work on gene regulation in more complex organisms. Yeast genetics also provided novel tools that subsequently became essential for genomics research in eukaryotes.
From the late 1980s onwards, several teams spearheaded fundamental developmental biology research. The teams led by Charles Babinet and Philippe Brûlet pioneered transgenesis and homologous recombination techniques in mice at the Institut Pasteur. Margaret Buckingham’s team also used genetically modified mice to investigate the role of structural and regulatory genes in skeletal and cardiac muscle development, with a particular focus on embryology. The group led by Jean-Louis Guénet used traditional mouse genetics techniques to identify the loci and genes responsible for specific phenotypes. Jean-François Nicolas and his team initially studied differentiation in embryonic carcinoma cells, then used innovative molecular tools to mark and monitor specific embryonic cell lines. Philip Avner’s laboratory led the way in identifying the mechanisms regulating X-chromosome inactivation and epigenetic regulation in mice. Together, these researchers explored fundamental questions in gene regulation and tissue organization. Their vital contributions helped consolidate a discipline that is still developing today.
Of flies, mice and men
At the Institut Pasteur the time had come at last to study development of the organism as a whole. Cell differentiation can only be understood if a study is carried out in vivo, in the organism as a whole, on the way cells interact and cooperate to form different tissues according to an established plan. This led to a surge in developmental research worldwide.
Firstly, since the start of the 1980s, thanks to the work of Martin Evans and his colleagues at the University of Cardiff (UK), it was now possible to extract stem cells from the embryos of healthy mice and culture them in their undifferentiated state. The team had even succeeded in genetically modifying stem cells to produce mice. These breakthroughs led to the development of transgenic mice—an effective tool for developmental biologists. Using this technique, Mario Capecchi from the University of Utah produced mice with a target gene deleted, or ‘knocked out’. Knockout mice soon became widespread in laboratories (homologous recombination technology) and earned their co-inventor Oliver Smithies the Nobel Prize in 2007, alongside Martin Evans.
Secondly, scientists had started to describe the genes involved in development. Edward Lewis at the California Institute of Technology, Christiane Nüsslein-Volhard and Eric Wieschaus from the European Molecular Biology Laboratory (EMBL) in Heidelberg (Germany) and their colleagues identified a number of genes in flies that play a vital role in development. This research, carried out at the end of the 1970s, received the Nobel Prize in 1995. In 1983, the teams of Walter Gehring, from the University of Basel (Switzerland), and David Hogness, from the University of Stanford (USA), isolated and characterized these genes, whose mutations were responsible for malformations that had been described in flies since the beginning of the 20th century. Homologs of these genes were subsequently found in other animal lines such as mice and humans, proving that the mechanisms involved had been well conserved in the animal kingdom.
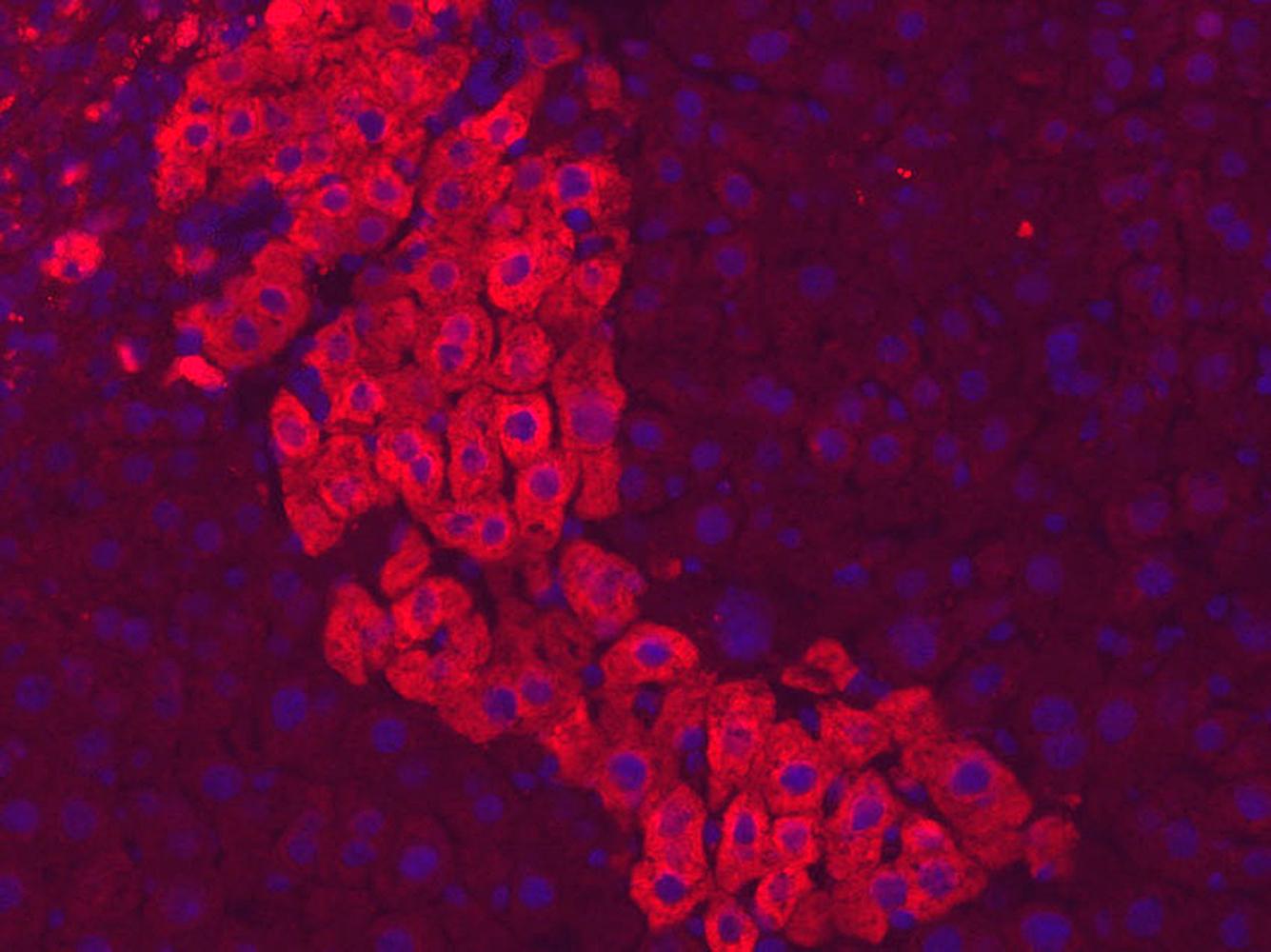
In addition, a fundamental discovery was made various teams, notably the team led by Takashi Matsui of Washington University in St. Louis (USA), showed that, in eukaryotes too, certain proteins play a part in the regulation of gene expression. Known as transcription factors, these are particularly instrumental in cell differentiation, guiding the DNA transcription machinery towards the genes to be transcribed into proteins. For each cell type to be derived from stem cells there is a defined cocktail of transcription factors that activate the expression of genes specifically for it. In the 1990s-2000s, hundreds of transcription factors were identified as associated with a particular tissue type—bone, skin, cartilage, muscle, etc. The Institut Pasteur played a part in this characterization process. Particularly worthy of note in the 1990s was the work of Moshe Yaniv, Mary Weiss and their colleagues, who showed that these proteins regulate liver cell differentiation in mice.
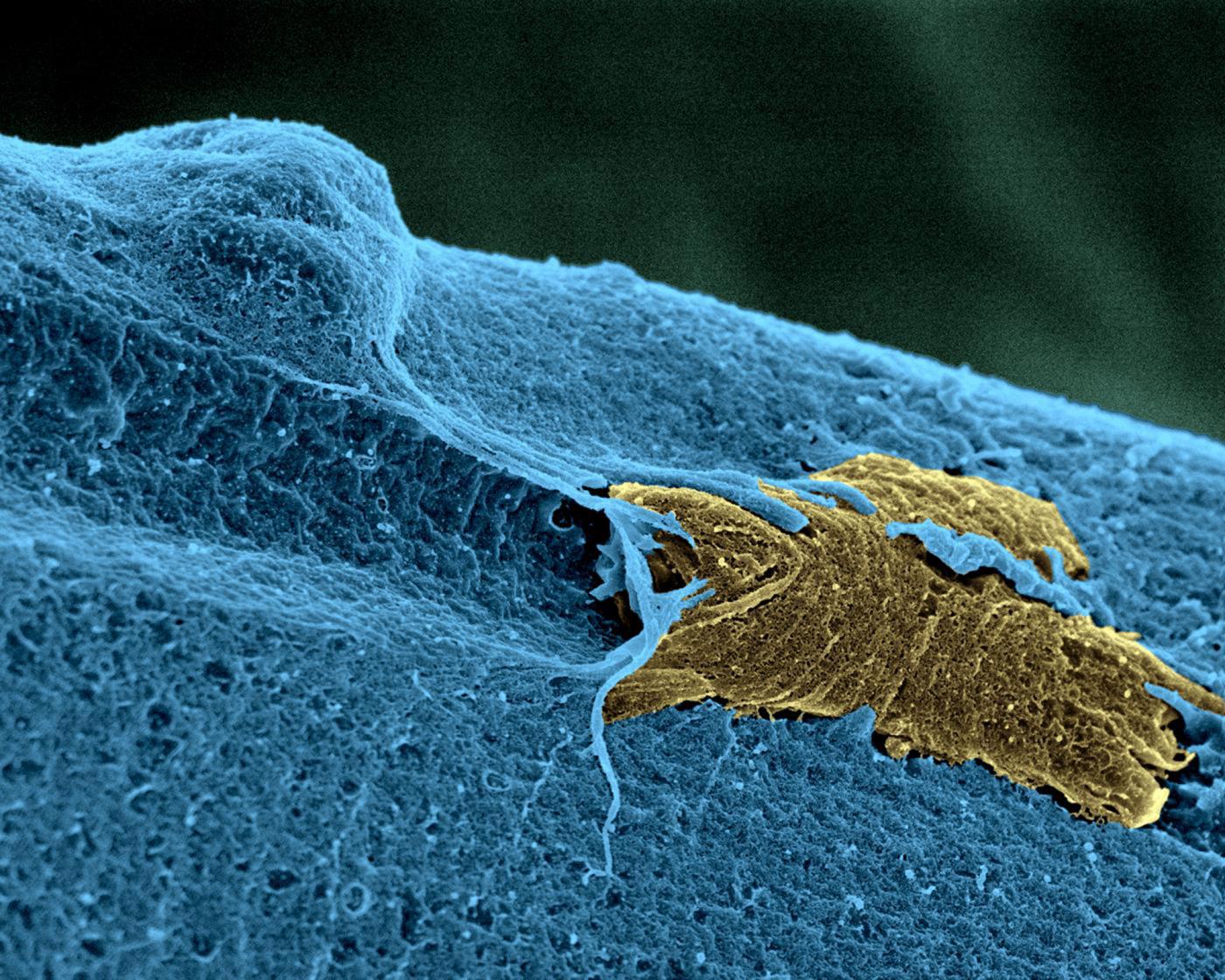
A skeletal muscle stem cell (in yellow), in activation state, on a muscle fiber (in blue). © Institut Pasteur/Unité d'Histopathologie humaine et modèles animaux - Plate-Forme Microscopie Ultrastructurale. Colorisation Jean-Marc Panaud.
Skeletal muscle stem cells
Shahragim Tajbakhsh and his colleagues identified a population of muscle stem cells that were in a more quiescent, or dormant, state than the others but capable of becoming active in the event of injury—a sign that they can change their metabolism. In collaboration with Fabrice Chrétien’s team, the groups showed in both mice and humans that these cells can survive for more than two weeks post mortem and retain their regenerative potential.
Studying stem cells in their own right
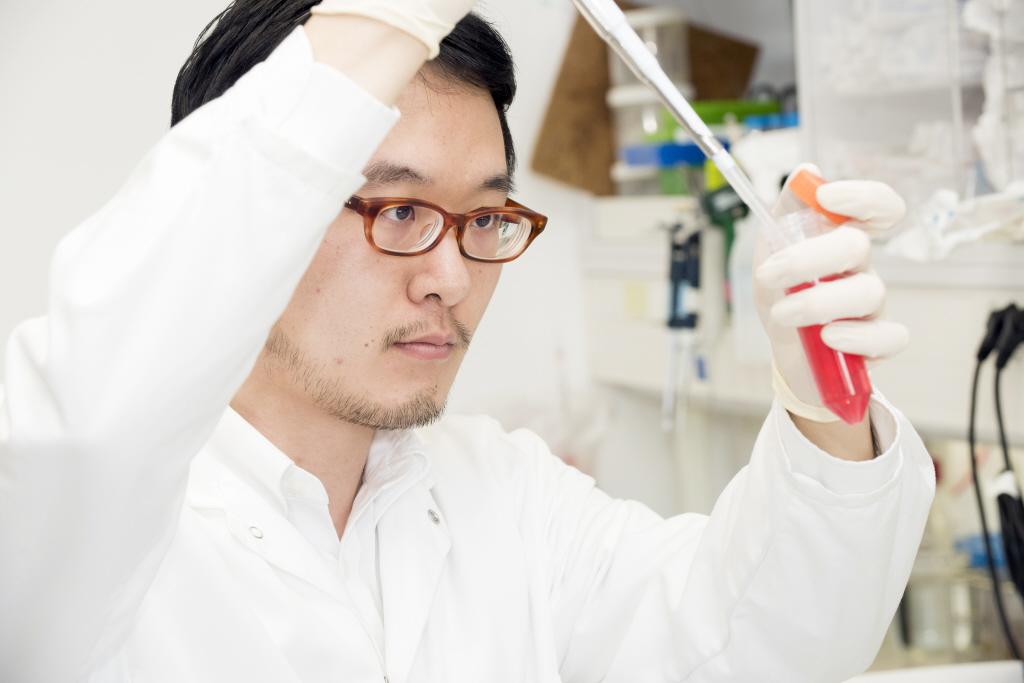
We now know both how to differentiate a stem cell into any cell type and to restore a differentiated cell to a pluripotent state. The technique for producing “induced” pluripotent stem cells (iPS cells) is much simpler to implement than transferring a cell nucleus into an oocyte (the technique used to clone Dolly the sheep in 1997), and was developed by Shinya Yamanaka of Kyoto University (Japan) in 2006, and rewarded by the Nobel Prize in 2012. Biologists also have access to tens of thousands of modified mice, some carrying several genes mutated in several places or with a deleted gene. It is also possible to produce fluorescent mice: a gene of interest is simply tagged with a jellyfish gene coding for a fluorescent protein. When this sequence is expressed it produces a protein carrying a fluorescent marker that shows up under the microscope—during embryogenesis, for example–and in the living organism.
Biologists work with all these tools, not only to identify the mechanisms at play during embryo development, but also to achieve more accurate characterization of stem cells themselves.
Generally, it is vital to make sure that pluripotent cells are eliminated, to avoid the formation of tumors. It is also important to determine whether these artificially produced pluripotent stem cells have the same functional potential as embryonic stem cells. As long as this question remains unanswered it is unlikely that they will be used in cell therapy. However, this would solve an ethical dilemma, given that embryonic stem cells are taken from surplus embryos that were frozen following in vitro fertilization for infertility. Induced pluripotent stem cells would be produced from the patient’s own tissues, and so also help to reduce transplant rejection.
The promise of stem cell research
This work has a number of important goals:
- to gain an understanding of the fate of embryonic stem cells,
- to gain an understanding of the formation of tissues and organs,
- to explore the biology of stem cells in the hope of using them in regenerative medicine for diseases such as Parkinson’s, Alzheimer’s, diabetes, certain muscle disorders or retinal diseases such as ARMD (age-related macular degeneration)—which are all associated with progressive cell death.
Clinical trials are under way to treat ARMD patients: a few skin cells are taken and reprogrammed as induced pluripotent stem cells; the cells are then differentiated into retinal precursor cells and grafted onto the patient’s eye. The initial results are promising, but it will be a while before the technique can be used routinely.
The abilities of stem cells
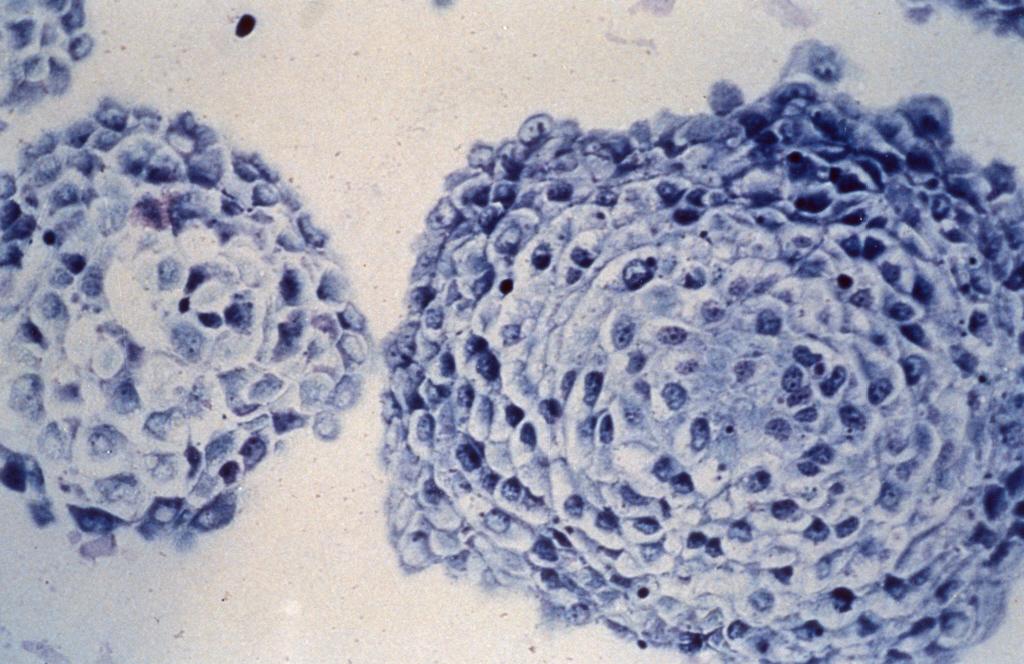
A major advantage of using a variety of model organisms is that scientists can focus on the salient features of each. François Schweisguth’s team, for example, has used live-cell imaging of sensory organ precursor cells in flies to examine cell fate determination in space and time. This is not a typical stem cell system involving self-renewal; instead, several rounds of asymmetric cell division yield multiple cell types including a neuron and associated cells. A number of regulatory factors including Notch and Numb are involved in these asymmetric cell divisions. This fundamental research provides critical insights into asymmetric cell division, a mechanism used by stem cells in many organisms to generate a variety of cell fates and to self-renew.
Pablo Navarro Gil and his team are attempting to identify the mechanism that enables pluripotent stem cells to be passed from one generation to the next. Embryonic stem cells renew themselves—in other words some of them generate new stem cells to form a cell reservoir.
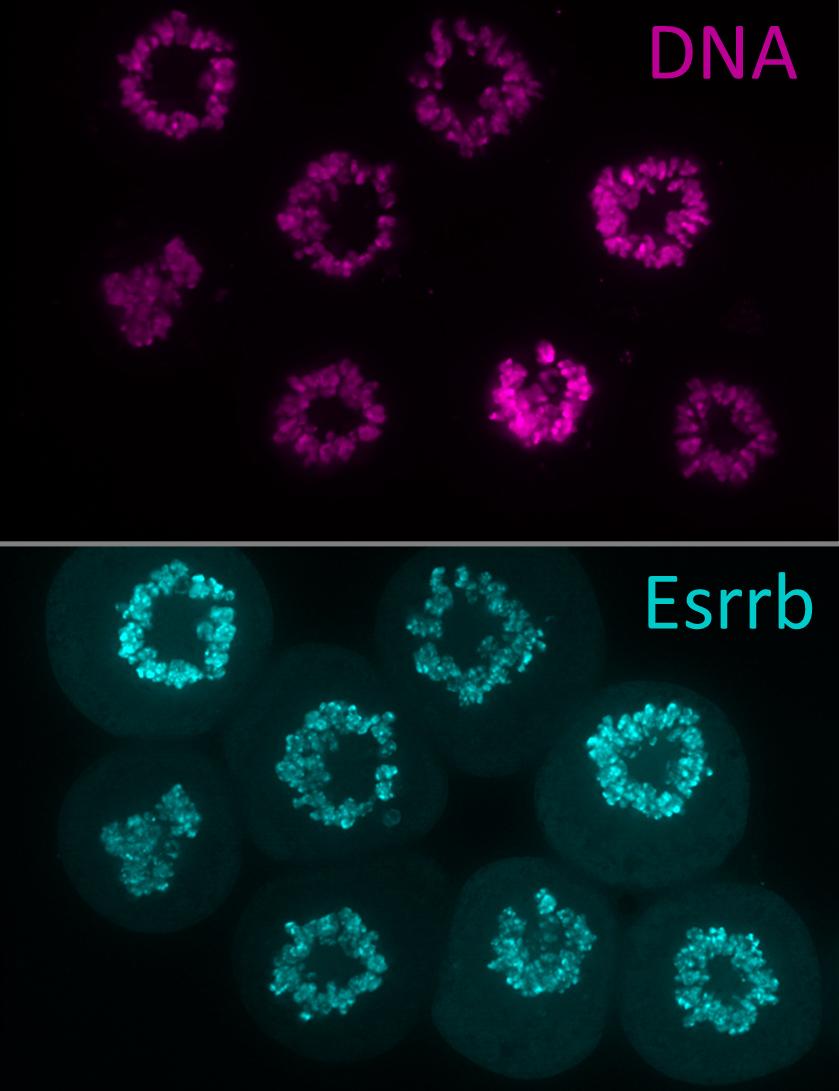
It is thanks to these cell reservoirs that some animals, such as lizards and salamanders, are able to regenerate limbs even in adulthood. Mammals, however, are not so lucky, and must repair any injuries they incur. Adult mammals retain some stem cells, but most of these lose their regenerative potential with age. For example, hematopoietic stem cells residing in the bone marrow continually renew red blood cells, which have a short life span. Used for over fifty years to help treat certain blood and immune system disorders, the stem cells are transplanted by IV injection and migrate to the host’s bone marrow, where they differentiate correctly. Biologists are trying to unlock the secrets of these stem cells: What is their role? How does aging affect them? Could they be reactivated?
During the last few years, the team led by Philippe Herbomel within this Institut Pasteur department used zebrafish to identify the niche where hematopoietic stem cells are produced. Using a molecular tracer he was able to monitor in vivo how these cells emerge in the animal model.
Although stem cell research is a major focus of interest at the Institut Pasteur, it is not an end in itself, as illustrated by this brief overview of the changing nature of research topics in what is now known as the Department of Developmental and Stem Cell Biology. Understanding the fundamental principles of gene regulation and cell fate involves a wide variety of different disciplines, including epigenetics, epigenomics, biophysics, single-cell analysis and real-time imaging, all of which converge to shed light on how interactions between cells result in organ morphogenesis during growth, regeneration, illness and aging. The major strength of this department is its sheer diversity— from the wide range of model organisms (flies, worms, zebrafish, mice and birds) and sophisticated techniques it uses to its multiscale research at molecular, cell and tissue level. The recent recruitment of a new generation of talented leaders with expertise in all these fields will further consolidate this diversity.
These are only a few of the research areas being investigated in this field at the Institut Pasteur. François Jacob and Jacques Monod could not have imagined a brighter future for their molecular biology department!
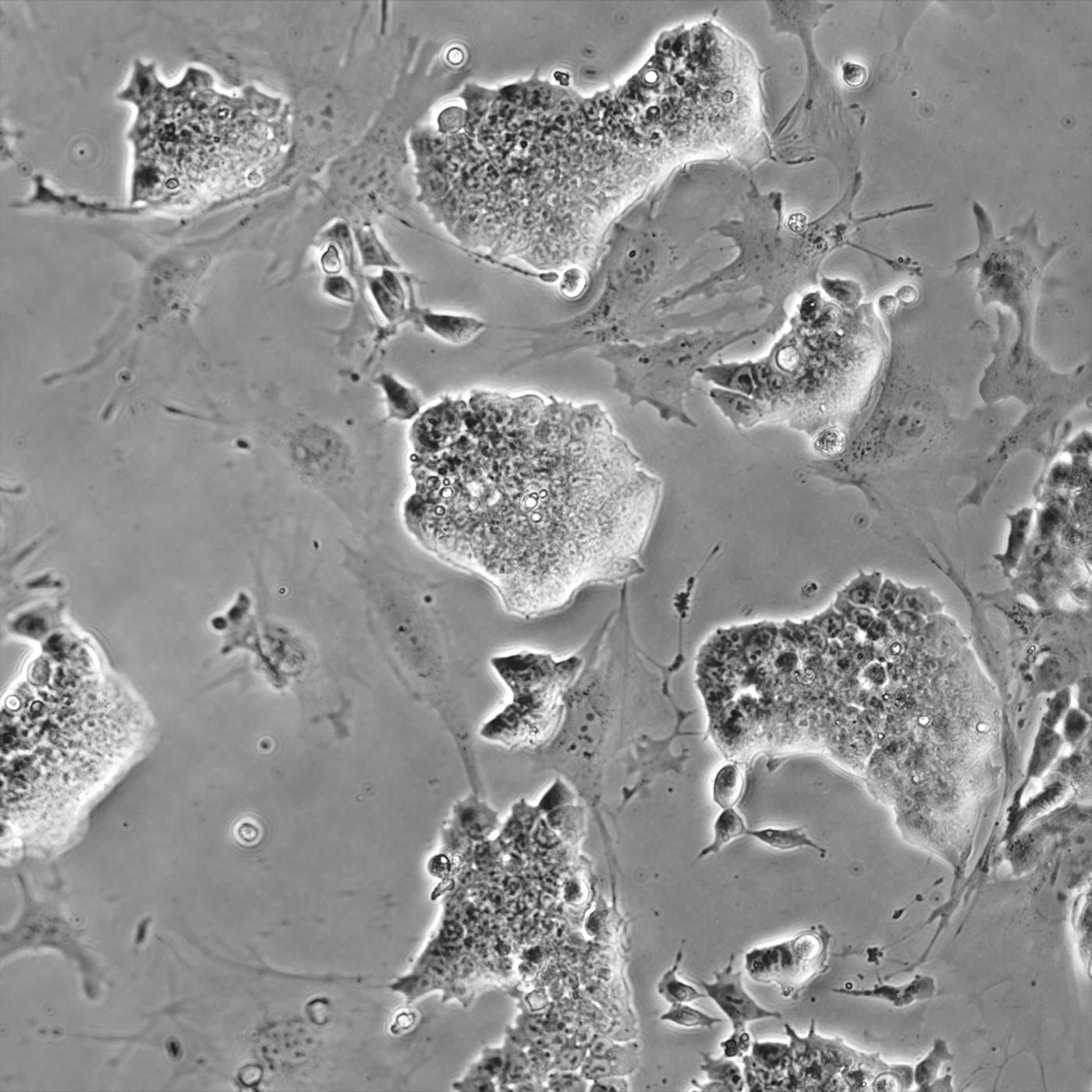
Embryonic stem cell colonies with rare cells isolated during spontaneous differentiation. © Institut Pasteur/Alexandra Tachtsidi
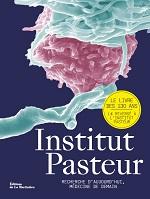
The Institut Pasteur thanks the set of people that has produced this book.